Steam, water, and ice are familiar to us — in the vapor rising from a hot kettle, a healthy spring rainfall, and the smooth surface of a skating rink. But to some materials scientists, these states of matter are more than mere changes in physical properties. They pave a path to new frontiers of computer science and data retrieval.
Researchers at the Paul Scherrer Institute recently discovered a method of organizing magnetic metamaterials so that they have phase transitions — walking the line between solid and liquid. This significant technological development, which draws on an elementary understanding of solid, liquid, and gas, could lead to better information storage.
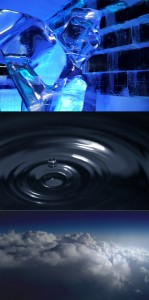
The team, led by Laura Heyderman, examined phase shifts in magnetic metamaterials — composite materials not found in nature that can be created to interact with magnetic fields. In their research, Heyderman and her colleagues tirelessly assembled one billion tiny magnets to create a honeycomb structure only five by five millimeters in size. This structure is unique in how it transfers magnetic information across the entire honeycomb. Then, the scientists subjected this layer of magnetic metamaterial to different temperatures, observing how it reacted by arranging its magnetic poles in different ways. As temperature decreased, the poles adopted formations that were more efficient in moving magnetic signals through the material. This phenomenon is not so different from the way that water molecules bind more tightly together when frozen into ice.
Phase shifting magnetic metamaterials like the ones produced by Heyderman’s team are become more important as scientists reach the limits of what conventional materials are able to accomplish in electronics. Recently, metamaterials became a hot topic in popular physics, when buzz surfaced over the creation of “invisibility cloaks.” Of course, there is some discrepancy between what is happening in the labs and what goes on at Hogwarts. The real world invisibility cloak carefully manipulates magnetic properties so that light can deflect around the hidden object instead of reflecting back to the viewer. Metamaterials have a variety of other potential applications, from photography color filters that actually alter light wavelengths to more powerful telescopes.
A huge potential market for metamaterials is nested in Silicon Valley, where researchers at microprocessor corporations are quickly running into difficulties as they try to build smaller computers. In the 1970s, researchers predicted that computational power would double every 18 months onward, an observation known as Moore’s law. To achieve this progress, the law actually anticipated that engineers would be able to make computer components smaller by a factor of two every 18 months. If transistors, for example, could be built small enough that twice as many would fit into the same computer, the machine would run twice as fast. However, there is a practical limit to how far scientists can shrink the size of computer pieces. As current technology stands, within a few generations, Moore’s law could be a dream from the past.
Magnetic metamaterials could help resolve the Moore’s law dilemma, especially as these materials relate to engineers’ current efforts to store information using the spin of an electron rather than its charge. This field of science is called spintronics. Modern electronics rely on the movement of electrons to store and retrieve information: As electrons move, their charges are measured and translated into computer bits. Billions of these bits are then used to load up websites and applications. Spintronics takes a different approach, measuring the electron’s spin instead of its charge. An electron’s spin, associated with magnetism, takes only one of two directions — up or down. If scientists can learn to manipulate electron spins, then this information could be translated into a more efficient computer bit.
Heyderman’s research could help scientists master the art of spintronics because it improves upon current understanding of how electrons share magnetic information. Her team’s primary discovery is that nanomagnets communicate more quickly with one another at low temperatures than at higher temperatures. To visualize nanomagnets’ increased efficiency at lower temperatures, you can think of how much harder it is to push liquid water than it is to push a block of ice. When you push the ice, the entire block moves forwards immediately. However, when you try to push on a pool of water, it does not simply move forward. Instead, you send ripples through the liquid, and it takes a much longer time for your push to reach the other end of the pool.
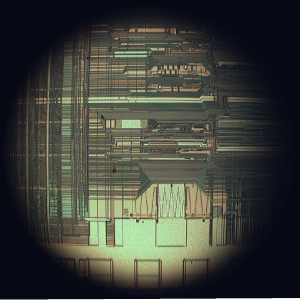
Magnetic metamaterials behave in a similar fashion, but rely on a magnetic signal instead of a physical push. At higher temperatures, the honeycomb of nanomagnets allows the signal to slowly ripple through the entire grid. In contrast, at lower temperatures, the magnetic metamaterials react more like ice, and even nanomagnets far away from the signal’s initial push respond almost instantaneously. If utilized in a computer chip, these magnetic metamaterials and signals could speed up information transmission.
Even with these accomplishments, Heyderman’s work is not complete. Her group still needs to experiment with metamaterials of different magnet arrangements and sizes, as small changes in arrangement could have big influences on large-scale magnetic and spin effects. So far, the experimenters have tested the effect with two different configurations of nanomagnets, each with different magnetic properties. For the weakly interacting sample of nanomagnets, the phase transition effect was almost non-existent until temperatures reached approximately 10 Kelvin. However, for the strongly interacting sample, the first signs of phase transition began at almost 145 Kelvin, a much higher temperature. The group will continue to test different arrangements of magnetic metamaterials, hoping to exercise even greater control over these phase transitions.
It is likely that we will see improved spintronics in the computer world within the next few decades, and consequently, faster computers. And yet, magnetic metamaterials retain an exciting similarity to nature’s own phase-changing products. The ancient ideas of phase transitions, from simple ice, water, and vapor, continue to spark scientists’ imaginations and promise new technologies to enrich our world.