Stick your hand into a tub of electric blue Play-Doh, and the rubbery clay gives way immediately, conforming to your fingers. As you lift out your hand, some of the moldable fun might still cling on! Believe it or not, the stickiness of Play-Doh is a close analogy to how the science of adhesion works. Sticky materials surround us; they are literally the glues holding everything together. They are in everyday products like Post-It notes, but they also guide science as diverse as the development of advanced glues and the biophysics of how cancer cells spread. Recently, a research collaboration led by Yale professor Eric Dufresne discovered a new kind of interaction between adhesive materials, paving the way for developing smarter sticky materials.
The concept of stickiness has been around for centuries, since early civilizations began repairing pottery with sticky tree resin. However, understanding why adhesives work took much longer. Even the creation of the Post-it note was an accident—its inventor was actually trying to create a strong glue. While those ubiquitous sticky notes arose from a fortunate accident, manufactures needed a better understanding of the underlying science to develop serious adhesives.
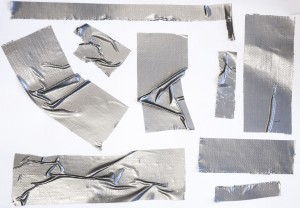
In the 20th century, researchers began to quantify the strength of sticky materials and come up with theories for why adhesion occurs. The most prominent model emerged in 1971, when researchers at Cambridge began to think of stickiness at the atomic level. Their idea was that all materials are deformable, so when two objects push against each other, both change their shape to a certain extent. This model can be thought of like the Play-Doh molding to your hand—if you only lightly pat the material, it does not have a chance to deform because the area of contact is too small. But when you really push into the Play-Doh, it spreads itself around your fingers, increasing the surface area of contact. Increased surface contact causes a larger frictional force, leading to stickiness.
This model dealt with a maximum of three different surfaces touching each other at a time: the two objects in contact and the surrounding air. While the model is logical, it has not worked well for advanced gel-like adhesives because these gels leak liquids, providing a new point of contact between the sticky materials.
Katharine Jensen, researcher at the Yale’s Soft Materials Lab, conducted a careful experiment exploring new causes of stickiness. Her team prepared two types of adhesive gels: one with liquid components and one without them. The researchers looked at how the gels stuck to solid objects at a micrometer scale, finding that water escaped from liquid adhesive gels as they deformed, similar to sponges. Liquid molecules have many different properties from solids, such as their ability to stick to each other by the property of surface tension. Therefore, the liquid component of certain materials changes the way that scientists calculate their stickiness.
But why is this discovery important? The old model works for many common adhesives, but the new research helps scientists better understand the stickiness of certain biological processes, like cancer cell mobility. Mutations in cancer cells transform the protein structure of their cell walls, leading to more deformable cell walls. Surprisingly, this causes cancerous cells to be more mobile than normal cells, allowing cancer to spread throughout the body more easily. This model seems to contradict past models of adhesion, since deformability typically increases stickiness. However, the new research may provide an explanation for why more deformability can lead to increased metastasis, the spread of cancer. The process of metastasis is complex, but these differences in adhesive properties are important to understand, as they may help researchers develop better detection methods for metastatic cancers.
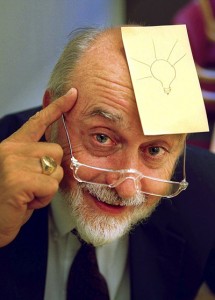
To be clear, this discovery does not contradict past theories of adhesion. Instead, it improves on the old model in an unprecedented way. Most adhesives do not leak water when squeezed, so the previous model is still a great approximation. The new theory also makes intuitive sense. “I’ve had a lot of people in the field say ‘I never would have thought it would have done that, but now that you’ve shown it, of course it does,’” Jensen told the Yale School of Engineering and Applied Sciences.
There are many potential uses for this new model, from improving regular synthetic adhesives to designing new types of gels. A particularly interesting development is in tissue engineering, as scientists seek to grow tissues for patients in need. Researchers currently use hydrogels as the template for growing tissues, as the cells stick to the gels to grow in the right shape. However, if the adhesion between the hydrogel and the cell is not exactly right, the produced organ could be a dud. This newly developed model could better tune the gel to the cell, reducing the number of duds produced.
Improved adhesives may soon surround us, but if they work properly, their presence will be undetected. They are the silent heroes holding our lives together. Improving our models of stickiness will guide the development of better adhesives, but perhaps the most significant takeaway from this study is how it exemplifies the incremental nature of scientific research. Instead of overturning previous models, new research improves our knowledge of the world bit by bit. Maybe that is the “stickiest” idea of all—science depends on steady research, improving old models in an ongoing search for truth.