What goes on in a cheetah’s brain when it hunts a gazelle? When a python strikes at a rabbit? When a bear snatches a fish from a river? When an orca attacks a seal? Innumerable predatory-prey relationships exist across the animal kingdom, yet little is known about how the brain controls predation. Which parts of the brain are involved? How does the brain coordinate the many muscles involved in hunting related tasks like pursuit and biting?
Researchers at the Yale School of Medicine, led by Ivan de Araujo, associate professor of Psychiatry, are looking for answers. The team of scientists identified a sub-region of the amygdala, an olive-shaped region of the brain, as the epicenter for predatory hunting. The amygdala has long been recognized as a contributor to emotions like fear and aggression, but these researchers dug deeper to understand the molecular pathways which drive predatory instincts. Shining flashes of laser light into the mice’s brains, the researchers could turn on or off the neurons that fire when mice hunt. Accordingly, the researchers could transform the mice at will from passive creatures to gluttonous predators. Through their findings, the team of scientists uncovered clues relating to the evolution of the brain.
Firing the Neurons
Previous studies showed that when rats hunt insects, the central amygdala (CeA), an almond-shaped sub-region of the amygdala, surges in activity. This led the researchers at Yale to question how the CeA of predators’ brains coordinate the plethora of muscles involved with hunting. “Predatory behavior is a particularly complex task because an animal needs a number of different muscles to run, jump on things, and use its head to kill,” De Araujo said.
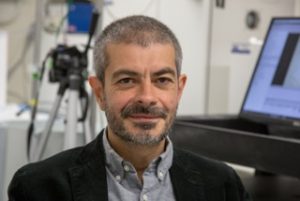
First, the researchers injected the central amygdala of the mice with a virus that contained a gene encoding a light-sensitive ion channel. Ion channels allow positively charged sodium ions to flow into the negatively charged axon of the neuron. The change in charge distribution along the length of the axon, called depolarization, causes the neuron to fire. Thus the virus made neurons in the CeA of the mice more prone to firing. The signal travels through the body until it reaches certain target muscles, such as the jaw and neck muscles.
To control when the neurons fired, the researchers inserted an optrode, an optic fiber cable connected to an electrode. Through this cable, the researchers could send a specific wavelength of laser light into the mice’s brains. This technique, called optogenetics, allowed the researchers to manually stimulate the parts of brains in mice which control hunting and biting by turning on or turning off the specific neurons involved when a mouse hunts using the energy from the laser.
“Optogenetics has two great advantages. First, you can target and study a specific group of neurons. Second, the control over when the neurons fire is specific. The technique is very transient and very fast,” said Wenfei Han, first author of the paper. Optogenetic activation triggered only the neurons injected with the virus to fire more intensely according to electromyogram (EMG) recordings, which measure electrical activity in muscles.
Initiating the Hunt
When the CeA was stimulated using optogenetics, the normally indifferent, docile mice transformed into bestial predators. When a cricket was placed in the cage, the mice captured and killed their prey in a much shorter time than they did when the CeA was not stimulated. The mice attacked even inanimate objects; when a bottle cap was placed in front of them, the mice grasped and bit the cap as if it were prey. Such attacks were not observed when the laser was off.
Upon laser stimulation, the mice attacked the closest object they could find. When a food pellet was placed in the opposite corner of the cage and the laser was turned on, some mice started to eat the cotton bed on which they were resting before stimulation. In some cases, the mice would grab onto the laser cable itself and chew straight through it. Unsurprisingly, when the cable split, the mice ceased hunting and returned to their previous docility. Even when no food was placed in the cage, the CeA-stimulated mice positioned themselves in an eccentric feeding position, tensing their hind legs and holding their front legs to their mouths as if they were eating. The researchers called this phenomenon “fictive feeding,” as it reflected the feeding position predators make when feasting on their prey.
These results showed that predatory behavior is an opportunistic behavior, meaning that animals hunt because they see the prey, not because they have a physiological need for food. Hunting opportunistically has an evolutionary advantage because if an animal waits until it is hungry, it may be too weak and likely die from exhaustion. “Opportunistic hunting may have remained in other species, including humans, because we also eat opportunistically. Our tendency to acquire calories even in the absence of hunger simply because we see food or see others eating reflects our fundamental hunting instincts,” De Araujo said.
The researchers also tested how mice interacted with each other upon laser stimulation. “When the mice were together, they became more curious, but we didn’t observe any attacks,” Han said. Interestingly, when a male and a female were placed in the same cage, the male started to groom the female. “Oral movements were triggered but not biting.” Han added. The researchers realized the amygdala was involved in many more complex behaviors than aggression and fear.
Digging Deeper
The researchers identified two distinct neural pathways critical for hunting, both of which originate in the CeA: one for pursuing prey, the other for biting. The neurons related to biting prey were located in the parvocellular reticular formation (PCRt), a region of the brain located just downstream from the CeA. When the CeA to PCRt pathway was stimulated, the mice stopped moving, sat down, and situated themselves in “fictive-feeding” positions. When the researchers disrupted the pathway with a pathway-specific lesion, the mice had a much harder time capturing their prey. The researchers reasoned that the neurons in the CeA interact with nearby neurons in the PCRt, enablingbiting activity by disinhibiting the circuitry in the PCRt. Thus, they concluded that the PCRt was dependent on the CeA and critical for delivering bites to prey, but separate from the pathway involved in pursuing prey.
For that task of pursuing prey, the researchers identified another region: the periaqueductal gray matter (PAG). The PAG, located in the midbrain, is typically associated with processing of pain. Activation of the pathway connecting the CeA and PAG decreased the speed at which the mice pursued their prey, decreased the time it took for the mice to initiate the chase, and decreased total hunting time. Lesions to this pathway caused a ten-fold increase in the delays to initiate pursuit. This delay was not observed when the CeA to PRCt pathway was disrupted. Further, activation of this pathway did not show fictive-feeding as did the CeA to PCRt pathway.
From their analysis of these pathways, the researchers concluded that the CeA acts as a control center, coordinating various, independent pathways in the brain related to effective and efficient hunting. “One way the brain may coordinates the different parts of the body involved in hunting is by positioning these two subpopulations of neurons close enough to each other that they can communicate and understand what the other is doing, yet projecting these two groups into different promotor areas of the brain so that messages to the legs do not get mixed up with messages to the jaw,” De Araujo said. Scientists have long sought this model for how the CeA functions and integrate processes related to pursuit with processes related to prey capture.
Evolutionary Ties
The emergence of gnathostomes, or jawed vertebrates, was revolutionary in the course of evolution. The success of jawed predators and the submission of jawless prey gave rise to a food chain with the predators on top. The appearance of jaws in vertebrates was met with additional physiological changes, such as the appearance of additional support bones in the shoulder to support more robust neck muscles. “A new head appeared at some point containing a jaw, mandibles, and neck muscles — structures which allowed animals to catch prey with improved efficiently,” De Araujo said.
Some hypothesized that jaws first evolved in fish for respiratory capabilities, contributing to gill bars, support structures that allow fish to breath. Opponents to this theory point to the morphological differences between jawed fish and gill bars. Regardless, the appearance of jaws ushered in new ways to obtain and process food, leading to a major diversification of species.
Furthermore, the rise of jawed vertebrates coincided with a major rewiring of the brain. One possibility is that the amygdala emerged as a command center for hunting activity. De Araujo speculates that the CeA appeared in vertebrates when the jaw and neck muscles appeared. Evidence for this hypothesis lies in one of few jawless animals left — lampreys. Genetic markers for neurons in the CeA are absent in lampreys. “That’s an indication that the major changes in the brain of vertebrates when their heads changed was the appearance of the central amygdala, because anatomical work on lampreys show that their brains look very similar to that of a reptile of a rodent,” De Araujo said.
Now with a new model for the role of the central amygdala and how sensory-motor tasks are achieved, these Yale researchers hope to use developmental genetic studies to determine the exact role the amygdala played in the evolutionary appearance of jaw and neck muscles. “Evolutionarily speaking, this work may help us understand what happened to the brain when the head sustained these major reconfigurations,” De Araujo said. The hunt is on for answers.
About the Author: William is a freshman Biophysics & Biochemistry major in Morse College. He is the copy editor for the Yale Scientific Magazine and works in Professor Forscher’s lab studying cytoskeletal dynamics underlying growth cone motility in neurons.
Acknowledgements: The author would like to thank Professor de Araujo and Wenfei Han for their passion and dedication to their research.
Further Reading: Han, W., Tellez, . . . Araujo, I. E. (2017). Integrated Control of Predatory Hunting by the Central Nucleus of the Amygdala. Cell, 168(1-2). doi:10.1016/j.cell.2016.12.027