How do our brains learn and memorize information, and where are memories located in our brains?
The neurobiological substrates of these processes have eluded neuroscientists for decades, but Yale’s researchers are shedding light on the mechanisms by which our brains remember through behavioral neuroscience research exploring emotional memory processing in rats.
Learning and memory are typically studied as one subject because they are mutually dependent; learning occurs when information has been stored in memory. On a cellular level, learning depends upon synaptic plasticity, the ability of neurons to change the strength of their synaptic connections.
Memory formation is accompanied by a process called long term potentiation (LTP), which reinforces the communication between neurons by supporting the synthesis of new proteins and secretion of specific signaling molecules. As Thomas Brown, Professor of Psychology and Physiology, aptly observed, “memory is the changes in synapses—you are your synapses.”
The hippocampus is a region full of changing synaptic strengths and connectivities and is central to learning and memory. Specifically, the hippocampus is essential for factual and spatial memory and is thought to play a major role in memory consolidation. Along with the hippocampus, the amygdala is another center of memory processing in the brain and is mainly responsible for emotional reactions.
The study of the molecules and brain regions involved in learning and memory processing aids our understanding both of normal and abnormal cognition. In addition to identifying how our brains typically store and represent memories, many common neurological disorders are based on dysfunctions of the brain’s learning and memory systems.
These include illnesses ranging from Alzheimer’s Disease to Post-traumatic Stress Disorder (PTSD), making these pathways important for both basic research and clinical applications.
Learning to Love
Besides for the straightforward learning and storing of factual information, memory researchers are also opening up the window to our emotions.
An essential subset of learning and memory research deals with the formation of emotional associations with memories: learning to love, hate or fear something requires that there is an emotional aspect of the memory stored alongside the unemotional components.
The history of acquired associations starts with Pavlov in his initial experiments demonstrating classical conditioning, where dogs learned to associate a neutral auditory stimulus (a bell) with a stimulus that produces a specific response (food), which causes dogs to salivate.
The conditioned dogs eventually salivated in response to the bell alone as well as in response to actually receiving food, showing that their brains learned to associate the bell with being about to be fed.
Fear conditioning in rats took the same basic paradigm but instead used emotionally salient stimuli to study acquired emotion.
A tone would be paired with a shock, and the animals would eventually learn to associate the tone with the shock and to fear the tone. This type of conditioning relies mainly on the amygdala and the thalamus, the relay station for sensory information.
Glenn Schafe, Assistant Professor of Psychology, has set out to discover the molecular pathways involved in the formation and storage of emotional memories in the amygdala by combining different levels of analysis in his research, from the molecular to the behavioral level.
The amygdala is plays an important role in acquiring fear-related memories, but debate continues about whether the memories are physically stored in the amygdala or whether the plasticity of the amygdala in fear conditioning is a reflection of changes in other brain areas.
In a single experiment, Schafe tested the success of fear conditioning with behavioral measures, measured activity of neurons in the amygdala with electrophysiological recordings, and pharmacologically inhibited essential molecules in memory processing in the amygdala.
By bringing together these three complementary analyses of the same question, Schafe proved that the plasticity of neurons in the amygdala underlies the storage of memories in the amygdala, both introducing a new paradigm for memory research and identifying the amygdala as a locus of stored fear memories.
Memory processing in the cortex
Another piece of the memory puzzle is the exploration of other brain areas that help shape our emotional memories. Brown thought that using tones didn’t get at the heart of the associative learning that happens during fear conditioning in rats. Instead, he used rats’ own vocalizations as cues for fear conditioning.
“When I came into this field,” Brown remarked, “the state of affairs was that we knew a lot about emotional learning to cues like tones. The more I thought about it, the more I thought that was really unfortunate. I thought, let’s look at complex stimuli, the kind of acquired emotion where you need your cortex, realizing that nothing like a continuous tone is ever heard in nature.”
These ultrasonic vocalizations (USVs) are socially important signals that communicate positive and negative messages depending upon the frequency of vocalization and so constitute a much more complex stimulus than a simple tone.
Because a USV is more complex, Brown hypothesized that higher cortical regions would be necessary to form the emotional associations underlying fear conditioning. In particular, he was interested in the perirhinal cortex, which is located in the temporal lobe, an important area for speech, memory, hearing, and emotional responses. Rats either with or without lesions to this area were conditioned by pairing a small shock with either a USV or a tone.
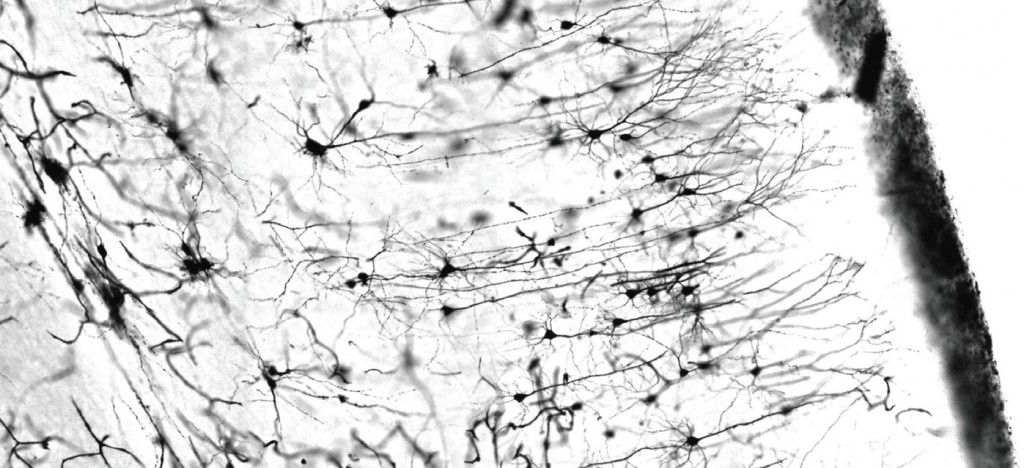
Conditioning to tones remained unaffected in the lesioned rats, but conditioning to the USVs was severely impaired, indicating a significant role of the perirhinal cortex in forming emotional associations with complex stimuli and identifying what is necessary to make conditioning cortex dependent.
The perirhinal cortex also integrates abstract sensory information from all of the brain’s sensory regions, connecting the hippocampus and amygdala and synthesizing many streams of already highly processed information.
Brown stated, “It’s polymodal—all of your sense are represented there in some of the most abstract ways, and for that reason alone it seemed like that’s the kind of structure you’d like to build emotional memories around: rich, multidimensional, and precise. You wouldn’t want to be scared of human faces in general, just a particular face.”
In humans, the perirhinal cortex could be necessary for storing some of our most complex and specific emotional memories. It is also the first target of Alzheimer’s Disease, making it a prime target of study for human therapy.
Wax on, Wax off
Deciphering the specific contributions of different brain regions to memory is a formidable task, as most of the ways to test the regions’ functions are either indirect or introduce new variables.
Creating lesions in brain can cause nonspecific damage to other essential areas, and imaging techniques like fMRI only show increased or decreased brain activity but don’t explain what the activity means.
To get around this problem, Brown pioneered innovations in a different technique that can be used to confirm the contributions of precise regions to processing—reversible inactivation.
Reversible inactivation using drugs avoids the problems associated with other methods because a region can be shut off, experiments performed, and then the same experiments can be performed once the region’s activity is turned back on.
This gives a perfect comparison of what the animal was capable of with and without the activity of this region. Historically, the downside was that researchers never quite knew where the drug went in the brain because drugs can diffuse away from the original injection site.
However, the Brown lab was the first to use a fluorescent version of an inactivating drug to circumvent this problem. They fused muscimol, a drug that suppresses neurological activity, to a fluorescent molecule called a fluorophore.
The result was fluorophore-conjugated muscimol (FCM), a fluorescent version of normal muscimol. The FCM enabled them to visualize the drug’s exact location in the brain with fluorescent microscopy because the fluorophore stays in the same location even after the drug is inactivated.
As expected, injecting FCM into amygdala significantly impaired rats’ normal behavioral response of freezing to stimuli they had been conditioned to fear. The same rats showed normal responses to fear conditioning after the FCM became inactive, demonstrating successful restoration of activity.
The breakthrough lies in the fact that they could analyze the rats’ brains to see where the drug had acted to produce the observed impairment in conditioning.
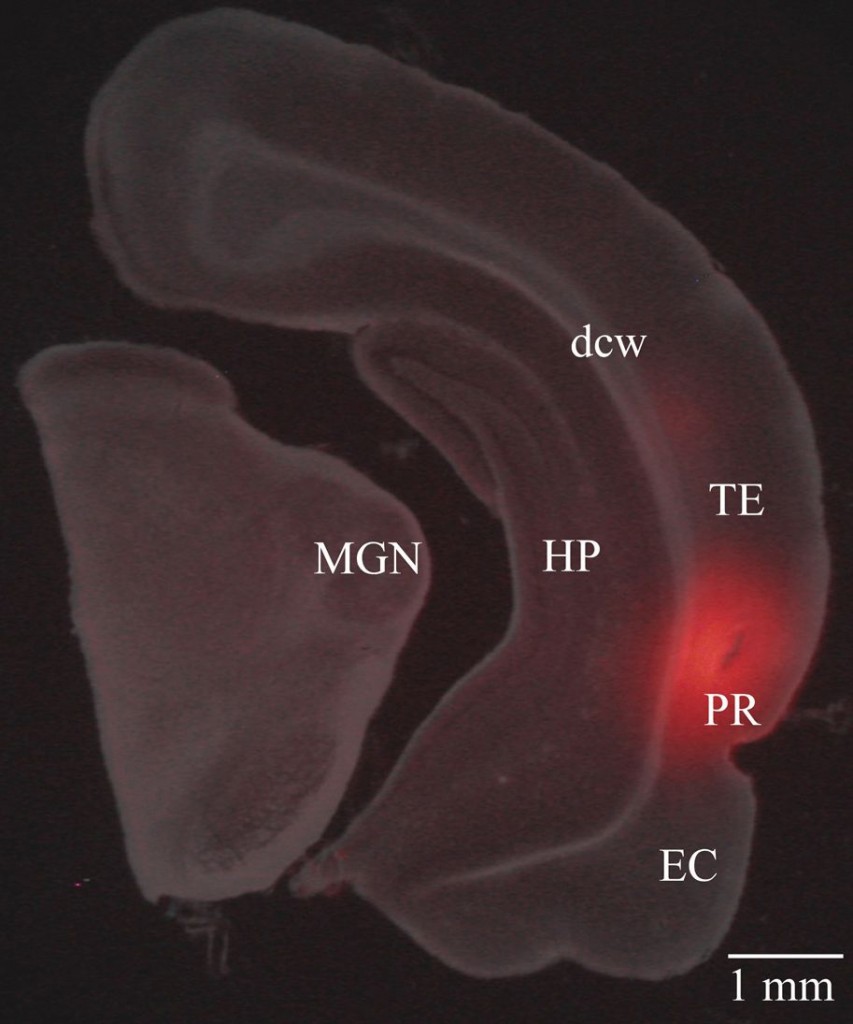
Memory in the future
The future is wide open for fluorophore conjugated drugs. FCM or other drugs can be used to investigate the functions of any small brain region, and the diffusion of drugs in the brain can be traced so that we know if they affect the regions they are supposed to target.
Brown expressed optimism about the applications of fluorophore conjugated drugs, predicting that “what we’re looking at in the future is all kinds of drugs with fluorophores to know exactly where they are.”
There is also enormous therapeutic potential in the identification of key molecules in fear memory processing. Psychological disorders such as posttraumatic stress disorder rely on the consolidation of emotionally laden memories that lead to significant psychological distress. Knowing the molecules and pathways involved in the storing of such memories creates new drug targets for treatment.
As Schafe explained, “everyone understands that traumatic events in one’s life lead to lifelong memories of that event, and in some cases, these memories are severe enough to lead to real psychological problems. Through understanding the neural mechanisms by which these are stored in the brain, we can begin to understand how to treat these psychological disorders.”
About the Author
ALEXANDRA PULST-KORENBERG is a senior psychology major in Ezra Stiles College. She continues to admire the amount we can learn about complex human behaviors from much simpler organisms.
Acknowledgments
The author would like to thank Dr. Brown and Dr. Schafe for their help in understanding the brains of our furry ancestors.
Further Reading
- Allen, T.A., Narayanan, N.S., Kholodar-Smith, D.B., Zhao, Y., Laubach, M., and Brown, T.H. 2008. Imaging the spread of reversible brain inactivations using fluorescent muscimol. Journal of Neuroscience Methods, 171(1): 30-8.
- Lindquist, D.H., Jarrard, L.E., and Brown, T.H. 2004. Perirhinal cortex supports delay fear conditioning to rat ultrasonic social signals. Journal of Neuroscience, 24(14): 3610-7.
- Schafe, G.E., Doyère, V., and LeDoux, J.E. 2005. Tracking the fear engram: the lateral amygdala is an essential locus of fear memory storage. J Neurosci. 2005 Oct 26;25(43): 10010-4.
- Nader, K., Schafe, G.E., and Le Doux, J.E. 2000. Fear memories require protein synthesis in the amygdala for reconsolidation after retrieval. Nature, 406(6797): 722-6.