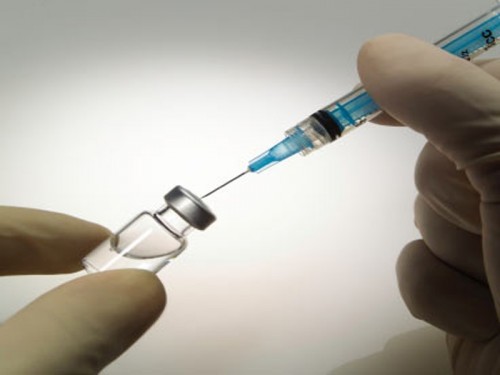
The human immunodeficiency virus (HIV) was first discovered in 1981. In the three decades since, HIV has become a household name and the HIV epidemic a top concern to scientists, doctors, and public health experts alike. This condition killed more than one million people in 2012. Once HIV enters the body, it systematically hijacks and destroys the helper T cells of the immune system. Victims are eventually left with almost no immune system function and thus largely susceptible to opportunistic infections. But how does the virus know which cells to target? Moreover, once HIV arrives at the cell, it cannot enter on its own, so what provides these signals and lets the virus in?

An Open Door: Chemokine Receptor Type 5
A few lucky people in the world are born immune to HIV. Upon investigation, researchers discovered that these people’s T cells have a non-functional allele of the gene coding for the C-C chemokine receptor type 5 (CCR5). In most people, CCR5 helps T cells react to chemical signals around it, but it turns out that the gene is redundant — humans without a functional copy of CCR5 still have normal immunological function. On the other hand, for a specific type of HIV, CCR5 is critical: the virus specifically invades cells displaying this receptor. Without it, HIV is locked outside the cell membrane.
These characteristics make CCR5 an excellent drug target, so a flurry of development began in the pharmaceutical industry. Companies such as Pfizer focus on small molecule drugs, which seek to either change CCR5’s conformation or to disrupt virus replication inside the cell. Unfortunately, several issues have arisen. First, some drugs require expensive tests to determine the type of HIV, and cost is a barrier to accessibility even in high-income countries. Second, the biological half-life of these drugs (a relative indicator of the amount of time that a drug is effective) is short. Because of this, patients must inconveniently take pills several times a day. If they forget, the outcome is worse than not taking the drugs, as HIV can develop resistance to the treatment. Third, many of these drugs are not well-tolerated. Finally, as only a few drug-resistant strains of HIV survive these medical treatments, all future generations of the virus will be unsusceptible to current medical treatments. Because of the issues surrounding traditional treatments, promising and novel strategies to combat HIV have emerged.

At the forefront of this new wave of research is Dr. Richard Sutton of the Yale School of Medicine. Sutton began studying HIV as a postdoctoral associate at the University of California, San Francisco. “I originally was studying a human retrovirus related to HIV, but when it became clear that HIV just wasn’t going to go away, I switched to studying that,” he explains. Sutton’s lab studies the mechanisms by which HIV replicates and then develops strategies to counter them. However, before human treatments can be deployed, animal models must be developed for testing.
Sutton’s lab has engineered such a model in mouse cells which has been partially successful. One of the most challenging aspects of developing a useful model is that HIV can only replicate efficiently in humans. One part of Sutton’s work centers on identifying genetic modifications that will make mice more susceptible to the virus. Transfecting mouse cells with the human chromosome maintenance region 1 (hCRM1) allows much greater production of HIV. hCRM1 is believed to contain regions important for exporting HIV’s RNA from the nucleus. This work may eventually lead to treatments and vaccines that can be tested on mice. Developing an immunocompetent mouse model would be a huge step forward for the field.
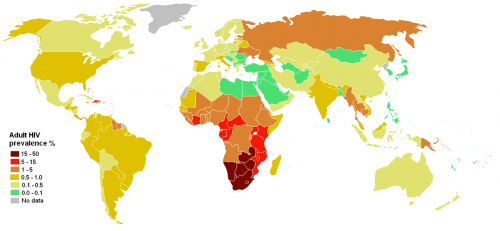
The War on HIV
“There are two major movements in the field: vaccination and eradication,” Sutton explains. So far, the vaccine branch of research has experienced little success. This struggle has caused renewed investigations into how patients develop immunity, or how patients “eradicate” HIV. If researchers can understand this process, vaccine implementation could be better targeted to elicit that specific response. One goal is to generate a broadly neutralizing antibody response, which could vanquish up to 90 percent of HIV isolates in the body.
The eradication movement is focused on CCR5 and other related receptors. If CCR5 could be removed in a safe and effective way, most strains of HIV would be left without an entrance point. However, the CCR5 protein itself is not the only target for treatment. In the central dogma of biology, DNA and RNA are used to synthesize the protein. Therefore, drugs can target different parts of the CCR5 synthesis process to achieve maximum effectiveness. Currently approved methods generally focus on changing the conformation — and thus, the behavior — of a key protein. Pfizer’s Maraviroc drug, for example, is a molecule that allosterically regulates CCR5 to change its conformation, making it unrecognizable to HIV.
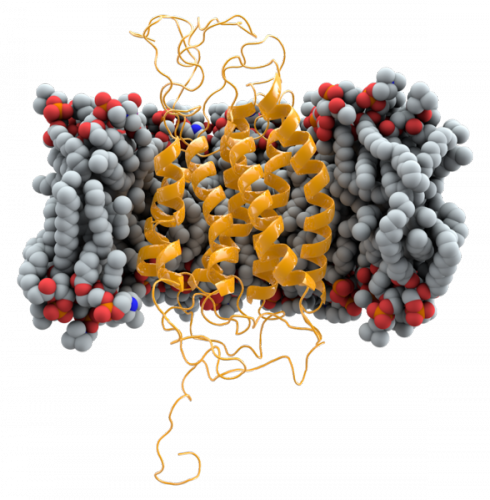
Recently, Dr. Dan DiMaio’s and Sutton’s labs discovered molecules that inhibit CCR5 expression. By testing several original proteins, these investigators identified certain sequences of amino acids that are complementary to CCR5’s shape in vivo, thus disrupting CCR5’s function, although the precise mechanism of action is unclear. Because these molecules are original, HIV has not yet had the chance to develop resistance to them. Therefore, the molecules worked well even against strains that were drug-resistant. These proteins also serve a second purpose in the study of how CCR5 is organized in the cell membrane. Such knowledge is incredibly valuable when developing new drugs.
Other research focuses on targeting HIV itself using a naturally occurring, dormant enzyme that breaks down HIV’s genetic material. By increasing the function of this inactive enzyme, researchers seek to prevent viral replication. An alternative strategy is to use molecules called small interfering RNAs (siRNAs), which target viral mRNA or CCR5 mRNA.
More permanent solutions that are currently under development involve the use of protein enzymes called nucleases. These enzymes cleave both strands of DNA. When the DNA is broken, the cell attempts to restore the molecule to its prior condition. The repairing process often introduces some sort of defect to the DNA. The deletion of one base pair, for example, causes a frameshift mutation. One codon is comprised of three adjacent base pairs, and each codon signifies one amino acid. Deleting only one base pair groups all subsequent base pairs into different codons, resulting in entirely different amino acids, and thus a different protein sequence and spatial conformation. The properties of this technique can be exploited on the human CCR5 gene. If the break occurs early enough in the sequence, the shape will be completely different. Therefore, HIV’s specifically-shaped proteins cannot bind to the altered CCR5 and cannot enter the cell.
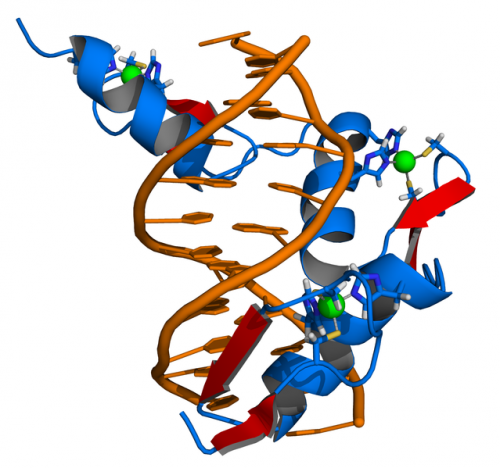
Looking Forward
The nuclease technique sounds ideal, and plenty of capable enzymes are available, so why not inject everyone with the enzymes? The main problem lies in specificity. As Sutton explains, “If you just put these enzymes into a cell by themselves, they could cut any region of DNA. If they decide to cut proto-oncogenes, then you are in trouble.” Proto-oncogenes are usually normal genes that are involved with cell cycle regulation, but if they suffer from certain mutations, the genes can lead to cancer. A secondary problem is effectiveness. Since the cell has two copies of every gene, both copies of CCR5 must be mutated, otherwise, CCR5 would still be expressed and provide an entry point for HIV.
Researchers are developing modifications to mitigate this problem. By attaching molecules that bind to specific DNA sequences, nucleases can be targeted to cut only at those locations. The three main strategies for DNA binding include (1) zinc fingers, which have shown efficacy in clinical trials, (2) transcription activator-like effectors, which use engineered molecules that also bind to DNA, and (3) a strategy based on the Archaea CRISPR system, which uses both a nuclease and small RNAs as guides. The last system is so promising that Sutton says, “If I had the time and resources, this is where I would invest them.”
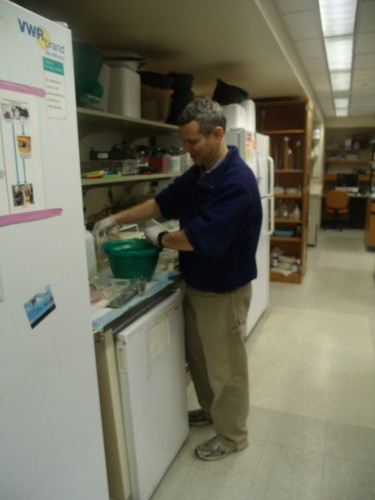
Despite the rapid progress of the field, Sutton says we still have a ways to go before a cure. More extensive clinical trials must be conducted for novel treatments, and side effects must be observed and considered. Before any method can be commercialized, treatments must be proven to be extremely effective in eliminating CCR5, and thus inhibiting HIV targeting. “Companies don’t get involved until they know there’s money,” he says with a chuckle. Though Sutton’s lab has identified drug candidates, an animal test model, and molecules to research, more substantive evidence must be provided for a drug’s efficacy and specificity before clinical trials can begin.
Even as Sutton’s lab and others continue to attack the problem of HIV from every angle in the hopes of finding a permanent solution, the quality of life for HIV patients continues to rise. An HIV is no longer the death sentence it once was, and through the efforts of researchers like Sutton, we are inching ever closer to an AIDS-free generation.
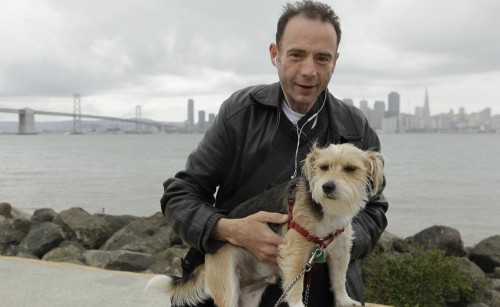
About the Author:
Kevin Boehm is a sophomore in Silliman College majoring in biomedical engineering. He is the vice president of the Yale Biomedical Engineering Society and conducts research in diagnostic radiology at the Yale School of Medicine.
Acknowledgements:
The author would like to thank Professor Sutton for taking the time to share his research and knowledge of HIV treatments.