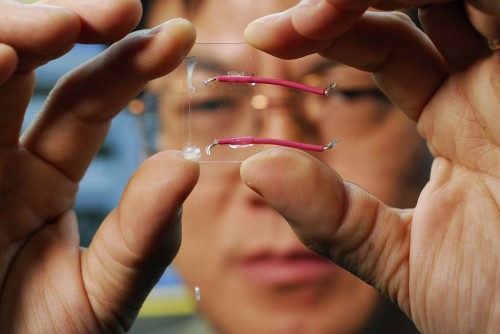
Energy exists all around us — in the motion of a heartbeat, the fluorescent light in an office building, and even the flow of blood cells through the body. These individual units of energy are relatively small, but they are numerous. Dr. Zhong Lin Wang, Professor of Materials Science and Engineering at the Georgia Institute of Technology, has developed a way to harness this ambient energy. After months of work, Wang and his team have developed the very first hybrid cell, which is capable of harnessing both motion and sunlight. By tapping into multiple sources of readily available energy, the tiny cells have the potential to revolutionize the way we power our devices.
All of our electronic devices, from medical sensors to calculators, require a constant supply of energy. Currently, the most common methods are a plug and power supply or batteries, both of which are large and thus limit miniaturization. Since Wang’s cell is small enough to work on the nanoscale, it can readily be incorporated into biomedical sensors, cellphones, and other small electronics. The cell’s hybrid design is an advantage as well: Solar energy alone produces high voltages but is unsuitable for devices used in the dark, while energy from ambient motion is more consistent but is available on a smaller scale. By combining these sources, Wang’s device can provide a highly reliable supply of electricity.
Wang developed the motion-harnessing component of the hybrid cell in 2006. These devices, called nanogenerators, can collect energy at the micro- and nanoscales of motion by relying on piezoelectricity, the production of a current from compression or strain. To construct a nanogenerator, Wang grew a vertical array of microscopic zinc oxide (ZnO) wires on a flat base. On top of this, he placed an electrode with multiple pointed peaks that give it a “zig-zag” appearance. When the ZnO nanowires are bent out of their ordered formation, they generate small electric charges due to piezoelectricity. They then touch the zig-zag edge of the electrode, which collects all the electricity to produce a current. Due to its sensitivity, a nanogenerator can capture even vibrations of very small magnitudes, which can then be harnessed to power an object such as a pacemaker. In fact, nearly a milliwatt of mechanical energy exists in each cubic centimeter of the ambient environment.

Many devices, however, cannot be sustainably powered by nanogenerators alone; solar cells generate a larger voltage more practical for use in bright environments. To miniaturize solar power capture, Wang made use of an existing technology called a dye-sensitized solar cell (DSSC). These cells are made by combining an anode with an electrolyte solution to form a semiconductor. First, a dye is applied to the anode to make it sensitive to light. When light strikes the dye, it releases electrons that flow through the anode toward the electrolyte solution, generating a current. Wang’s method employs the same principle on a miniaturized scale. Dye-coated ZnO nanowires serve as the anode, surrounded by the cell with a chamber of electrolytic fluid, forming a DSSC small enough to integrate with a nanogenerator.
After refining both technologies in collaboration with Dr. Xudong Wang of the University of Wisconsin-Madison, Wang has discovered a way to incorporate both nanogenerators and DSSCs into a device he terms a “hybrid cell.” The upper layer of the cell harvests light energy, and the nanogenerator below collects ambient motion. A single layer of silicon is sandwiched between the two and functions as an electrode for both devices, combining their energy into a single output. The two sources can be connected in parallel for higher currents and in series for higher voltages.
Even in the absence of light or motion, the circuit can still be completed. This is highly desirable because it generates electricity based on what is available. The hybrid cell captures what it can from the environment, but it is not limited by the absence of one source. Furthermore, although the nanogenerator alone produces a low voltage, combining it with the solar cell boosts the overall voltage of the device. These complementary sources allow the device to efficiently use energy resources in a variety of environments and situations.
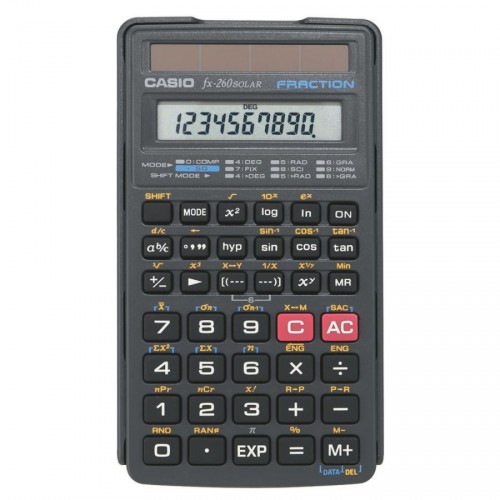
Hybrid energy harvesters are well suited to power implantable medical devices and other small electronics. In particular, Wang has proposed the installation of hybrid cells on sensing devices that gather information about the environment. This would replace traditional macroscopic sensing and provide more points of data for analysis. Using this richer data source could revolutionize fields such as environmental temperature studies, military reconnaissance, medical endoscopies, and underwater exploration.
However, there are many factors that must be addressed before this technology can be deemed dependable enough to power life-saving medical devices and other valuable electronics. One major problem is consistency, since solar energy cannot be harvested within an organism due to the lack of light. Additional complications arise from the ZnO wires in the nanogenerator. They are not all of the same length, resulting in some wires that are too short to touch the zig-zag electrode and others that are too long to flex and produce a current.
Wang and his team are working to address these challenges. To improve the nanogenerator component, Wang anticipates increasing the wire density to result in greater power output: If there are more wires per unit area of the substrate, there will be more electricity generated. Researchers are also investigating devices that can harness other sources of energy, such as thermal and chemical, and be incorporated into the cell. Biochemical energy — using enzymes to catalyze energy-yielding reactions — is particularly attractive due to its prevalence inside an organism where light energy is low.
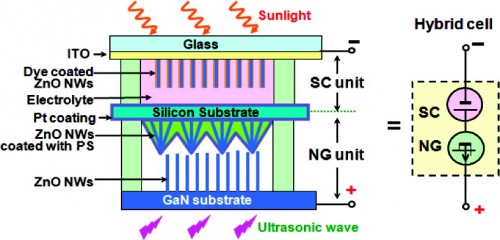
The integration of two energy-harnessing methods is the true genius of Wang’s work. As the movement for self-powered electronics gains momentum, future combinations may harness thermal, biochemical, and other energy sources depending on the device’s location. Each energy source has its own limitations, but integrating multiple collectors into one device leads to efficiency, reliability, and sustainability. It may not be so long before our iPods are powered by the steps we take in our morning jogs.
About the Author: Kevin Boehm is a sophomore in Silliman College majoring in Biomedical Engineering. He is the vice president of the Yale Biomedical Engineering Society and conducts research in diagnostic radiology at the Yale School of Medicine.