The DNA of the human genome contains a staggering three billion base pairs. Each of those base pairs is painstakingly replicated in each cell of the body. Most of the time, replication occurs without a hitch and a perfect copy is produced; because each cell’s DNA replicating machinery is so stunningly accurate in its work, it almost never makes a mistake. But when it does, even the tiniest error in copying the genetic code can lead to significant problems. A single point mutation – a mistake in copying just one of the billions of bases – can cause debilitating diseases such as cystic fibrosis, Huntington’s disease, muscular dystrophy, fragile X syndrome, and many more. Still more genetic diseases are caused by a combination of mutations in the genome.
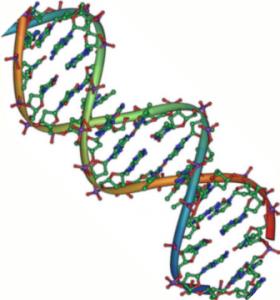
Rare Genetic Disease
The many small changes that can possibly occur in the genome amount to causing some 5,600 identified rare genetic diseases. These diseases affect around 24 million people in the United States alone and 240 million people worldwide. And alarmingly, despite the prevalence of these diseases and the substantial health problems they cause, only 5% of these diseases have an FDA approved drug treatment. Scientists at Yale are hoping to change that.
In order to tackle the large number of unsolved genetic disorder mysteries, scientists are combining the knowledge and methods of several different disciplines. The newest approach to this type of research uses genetic sequencing technologies to bridge the gap between basic science research and clinical application. By using two different approaches, scientists around the globe, with Yale researchers at the forefront, are striving to reach a new understanding of the mechanisms behind rare genetic diseases and to find potential applications for therapeutic drugs.
Yale Research
Two Yale scientists working at the intersection of research methodologies are Dr. Leonard Kaczmarek, who researches neural physiology, and Dr. Christopher Pittenger, who studies the biological causes of obsessive, repetitive behaviors. Both professors were working on basic science research when genetic sequencing implicated a gene in connection to a rare genetic disease. Their previous research enabled them to figure out and experiment with mutations in these respective genes. Describing these encounters as “out of the blue” and “lucky,” respectively, Kaczmarek and Pittenger have produced research that advances our understanding of these rare genetic diseases. As Kaczmarek’s and Pittenger’s stories show us, basic and clinical research can be linked to solving rare genetic diseases.
Epilepsy and Ion Channels
Kaczmarek worked in the area of neuropharmacology at the Yale School of Medicine. He conducted basic research on neuron firing, particularly on the role of ion channels. While researching neurons, his lab discovered a potassium channel, called the Slack potassium channel, which may play a role in neural plasticity and cognitive function. This channel is activated by high levels of sodium and is common throughout the central nervous system.
One day, Kaczmarek received an unexpected call from a French neurologist working in Paris. According to the neurologist, genetic sequencing had hinted at a particular mutation responsible for a rare disease called Malignant Migrating Partial Seizures in Infants (MMPSI); and more importantly for Kaczmarek, this mutation affected the Slack channel.
MMPSI is one of several types of epilepsy. It occurs in young infants, and often goes undetected because it does not cause infants to have the convulsions that typically accompany a seizure. It results in the arrest of cognitive and motor development at six months of age. The seizures eventually subside as the patient ages, but development remains at a standstill. Often in cases of the disease, parents bring their infants to a doctor not because of seizure symptoms, but because the infant fails to normally interact with the parent. In MMPSI, seizures occur in varying parts of the brain as electrical activity migrates.
The cause of MMPSI, as Kaczmarek discovered, is a mutation in the gene that codes for the Slack channel. This rare mutation causes an increase in the amount of potassium flowing through the Slack channels in an infant’s nervous system. The increased activity of the Slack channel results in the neuronal problems that characterize MMPSI. Kaczmarek was able to show that mice lacking the Slack gene could not complete procedural motor learning tasks. In this way, he demonstrated a link between genetically-induced overactivity in the Slack channel and the impairment of motor skills development. With Kaczmarek’s research, the Slack gene became the first gene clearly associated with epilepsy that was identified through exome sequencing (an approach that sequences select regions of the genome, as opposed to whole genome sequencing).
Despite the outstanding contribution he has made to medical knowledge and the potential for therapeutic treatment, Kaczmarek worked in basic research for most of his career as a scientist. “I was never primarily interested in diseases and I never thought I would be working directly on human mutations,” Kaczmarek said. Kaczmarek’s experience shows how advances in gene sequencing suggests are connecting basic science to clinical relevance in a new way as geneticists seek out and collaborate with the scientists studying basic mechanisms related to affected genes.
Tourette Syndrome and Mouse Models
Kaczmarek is not alone in his encounters with the potential of genetic sequencing. Christopher Pittenger, another Yale scientist, also found the connection between his clinical research and a particular rare genetic disease through genetic sequencing. Pittenger runs a neuropsychiatric laboratory at the Connecticut Mental Health Center at Yale. He studies inflexible repetitive behaviors such as nail biting, drug addiction, and OCD, and has a particular interest in brain circuitry involving the basal ganglia, a region of the brain involved in movement, procedural learning, and cognition. He works with mouse models to demonstrate psychiatric causes and effects.
Pittenger was working on the phenotypic behaviors of Tourette syndrome when the association of a gene with Tourette symptoms led to a new angle for working on this disease. Tourette syndrome is characterized by motor and vocal tics that are involuntary and repetitive, such as uncontrollable head movements or randomly shouted obscenities. These tics emerge when the patient is a child and continue on into adulthood, peaking in severity during the late teen years. The disease is hereditary, and disproportionately affects males.
Tourette’s is not caused by a single gene mutation; rather, it is a polygenic disorder that can be caused by multiple different mutations with a sort of aggregate risk caused by the right (or wrong) combination. Scientists believe that each mutation may affect a common biological pathway at a different point, but this hypothesis is yet to be confirmed.
Pittenger was introduced to a family suffering from Tourette syndrome and genetic sequencing identified a common mutated gene with a potential role in the pathology of the disease. Pittenger’s geneticist collaborator, Matthew State, found that genetic sequencing of this family’s DNA revealed a common mutation in a certain gene, called histidine decarboxylase, or Hdc. This particular enzyme is necessary for the synthesis of histamine, which is a compound produced when the body is mounting an immune response. Pittenger’s lab took the next step in investigating the disease by examining a mouse model with a mutation in the Hdc gene. He sought to prove that the link between the mutation and the disease was causative, and not merely correlative.
“Show me a mouse with spontaneous tic-like behaviors and I will be unconvinced that’s it’s really telling us anything about Tourette syndrome,” Pittenger said. “But begin with the mutation, and create a mouse with a tic – this basically proves that the mutation is linked to human disease.” As Pittenger discovered, mice that had their histidine decarboxylase gene knocked out did indeed exhibit tic-like movements. This finding confirmed that a mutation that hinders the function of the histidine decarboxylase gene is sufficient to lead to the development of Tourette syndrome. Furthermore, when the mouse brains were injected with histamine, the tics disappeared. The study confirmed that deficiencies in histamine levels contribute to the development of Tourette syndrome.
Other genes are currently being explored by Pittenger and other researchers in a search for an inclusive biological pathway that may explain this polygenic disorder.
Pittenger feels that genetic analysis provides a strong basis for clinical research since it can explain phenotypic behaviors. As a psychiatrist, he is motivated to work at the intersection of basic research and clinical studies in order to alleviate human suffering. “The clinical perspective shapes more well defined questions,” Pittenger said, “as we are always forced to ask ourselves: does this matter?” To Pittenger, the objective is to continually fill in the gap between “human suffering and the neurons of the mouse brain” in order to find new strategies for understanding and treating disease. Rare genetic disorders provide an opportunity to further our understanding of neurological impairments and conditions that can be confirmed and tested in mouse models.
New Technology
In light of these recent developments to combat rare genetic disease, Yale received an 11 million dollar grant from the National Institute of Health in 2011 to establish the Center for Mendelian Genomics at Yale. The center has the latest and most advanced technologies for exome and complete genome sequencing; one of them, a bench-top sequencer called the Ion Proton Sequencer, can sequence the entire human genome in under 24 hours. The objective of the new institute is to provide key resources for Yale researchers working on genetic diseases across all departments, ranging from Neurology to Cell Physiology. Faculty members hope to aid the discovery of mutations responsible for rare genetic diseases in order to improve diagnosis and eventually treatment. Now, instead of distant geneticists contacting Yale researchers, collaboration can be localized and generated within the Yale community. The new Center for Mendelian Genomics provides the genetic sequencing bridge between basic research and disease pathology with the potential for clinical application.
Future Work
With genetic sequencing technology at the fore, Yale researchers like Pittenger and Kaczmarek are well equipped to tackle the rare genetic diseases that have eluded understanding by the scientific community until now.
Yale is poised to make significant contributions in the crusade against rare genetic diseases through a combination of the latest technology and creative and impassioned scientists and clinicians. The link between a genetic mutation found through sequencing and basic research will no longer be a “lucky” find but rather the inevitable result of systematic collaboration. With a tremendous group effort by scientists, geneticists, and clinicians, Yale is well poised to lead the fight against rare genetic disorders, one mutation at a time.
Acknowledgments: The author would like to thank Dr. Christopher Pittenger and Dr. Leonard Kaczmarek for their time and dedication to the pursuit of scientific knowledge and clinical application.
About the Author: Theresa Oei is a junior in Pierson College majoring in Molecular Biophysics and Biochemistry. She works in Joan Steitz’s lab on discovering genomic targets of viral miRNAs.