In a humid room on George Street, amidst a chorus of rushing water, 20,000 zebrafish dart back and forth in hundreds of plastic tanks. Silvery yellow and no longer than an inch, they all look the same to the casual observer. Neon labels on each tank, however, reveal each colony’s secrets — young or old, male or female, some un-mutated, others with blood vessels that glow fluorescent green.
This is the research world of Dr. Stefania Nicoli, assistant professor of cardiology at the Yale School of Medicine. Using these fish as a model system, Nicoli studies how living things develop — what genetic pathways are involved in the course of proper development, and what happens when these pathways go awry. “We are particularly interested in neurovascular development, the interaction between neuronal and vascular cells in building the central nervous system,” Nicoli said.
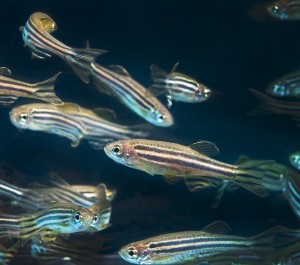
Nicoli and her colleagues recently uncovered a pathway that controls the timing of brain development. By interfering with one particular RNA molecule in zebrafish embryos, the team was able to throw off the timing of neuron production in the hindbrain. “What is clear,” Nicoli said, “is that there is a very tight regulation of this timing during neurogenesis to allow stratification of the neurons.” By examining this pathway, Nicoli and her colleagues hope to begin piecing together a detailed picture of brain development.
How to build a brain
The brain is built on a strict schedule. In humans, what starts as a handful of cells becomes a computational powerhouse capable of memories, emotions, dreams, and ideas. A dense, highly efficient network of 10 billion neurons is constructed in the course of only nine months. For proper development, timing is everything.
Nicoli’s project sheds light on a crucial piece of this schedule — the point at which the production of new neurons, a process called neurogenesis, comes to an end. “Every animal model has what’s called a neurogenic clock,” Nicoli said. “Neurons stratify in this cortical layer, and at some point they decide to stop.”
In normal zebrafish embryos, neurogenesis stops after a day and a half. In the experimental group of zebrafish, in which one specific RNA molecule was knocked out, Nicoli’s team found that this deadline was postponed. Without the activity of this key RNA molecule, the fish continued making neurons for six more hours, resulting in an unusually high neuron count. But the altered fish are not necessarily smarter. There is no link between neuron count and intelligence, Nicoli explained, adding that the extra neurons may have died during another step in development. For developmental biologists, the more alluring question involves the molecular trigger that led to this outcome — how can one pathway control neurogenesis so precisely?
A world run by microRNAs
At the heart of the pathway that Nicoli identified is a class of small RNA molecules called microRNAs. These were widely recognized by the scientific community only two decades ago, when they were discovered in the worm C. elegans. Although microRNAs have the same chemical makeup as messenger RNAs, their function is fundamentally different from that of their larger, better-known counterparts. MicroRNAs act more like interceptors than messengers: Whereas messenger RNAs shuttle genetic information from the nucleus to the ribosomes, carrying the vital instructions for protein synthesis, microRNAs are responsible for stopping them. They either destroy these messengers en route, or prevent their scripts from being read properly once they arrive at the ribosome.
It may seem paradoxical at first, but microRNAs play an important role in determining which pieces of the genetic code are expressed and which are silenced. And here in lies why they are so fascinating in developmental biology. All cells carry exactly the same DNA, but differences in microRNA expression between one cell and the next can profoundly affect a cell’s fate.
Previous work in this field showed that an enzyme called dicer is an important player in microRNA biogenesis. Dicer activates microRNA molecules, and therefore influences microRNA levels from cell to cell. But it turned out that dicer itself was not the master switch of the pathway identified by Nicoli’s team — governing dicer was actually another microRNA, called miR-107. “We performed several experiments where we showed that miR-107 specifically binds and regulates dicer,” said Emma Ristori, a postgraduate associate and the lead author on the study. By controlling this key enzyme behind the scenes, miR-107 can indirectly control other microRNA levels.
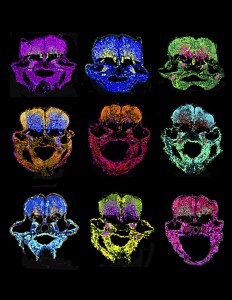
Nicoli’s team determined that levels of one particular microRNA, called miR-9, were especially sensitive to this chain of events. “We looked at the brain-enriched microRNAs, and found that not all of them were affected by this interaction [between dicer and miR-107],” Ristori said. “And miR-9 is a neural microRNA — very important for the specification of neurons and for neurodevelopment in general,” she added.
Specifically, Ristori observed that when miR-107 activity is blocked, dicer activity jumps up, and more miR-9 is converted to its active state. Ultimately, this then results in a longer bout of neuron production, as seen in the zebrafish designated to the experimental group. Conversely, cells with enhanced miR-107 see that dicer churns out less active miR-9, and fewer neurons are produced in the developing brain. Thus a single molecule like miR-107 has the ability to throw the neurogenic clock off kilter by regulating another microRNA and altering gene expression.
Nicoli believes that this pathway, and particularly the expression of miR-107, plays a key role in coordinating brain development. In fact, not all regions of the brain are producing neurons at the same time — this is because miR-107 levels across cells can vary depending on the region and stage of development. Much like sheet music in an orchestra, miR-107 delivers slightly different instructions to each group of nerve cells. As a result, this microRNA can dictate when different sections of the brain start and stop producing neurons.
Lingering questions
Though their project sheds light on a critical pathway in brain development, Nicoli and Ristori point out that a number of unknowns remain. For instance, though most fish with blocked miR-107 only had higher neuron counts, a few also suffered from heavy brain bleeding. The team has yet to explain these unusual hemorrhages. “A future project could investigate this connection between the neuronal and vascular cells,” Ristori said. “The crosstalk between vasculature and neurons is known, but we have to understand what the defect is [that leads to hemorrhaging.]”
Another area of future study is the impact of this pathway in treating neurological disease. Researchers have seen irregular miR-107 expression in both Alzheimer’s and schizophrenia patients, but the patterns are inconsistent. “In schizophrenia, they found an upregulation of miR-107, while in Alzheimer’s they found a downregulation,” Ristori said. Although miR-107 does seem to be a piece of the brain development puzzle, its role in these complex diseases has yet to be fully explained.
Nicoli plans to continue exploring not only miR-107 and its functions, but the entire microRNA world. “MicroRNA was discovered quite a while ago, but the way that it works is still a little bit mysterious,” she said.
Of course microRNAs do not give up their secrets easily. For one, they work as a team — even if we knock out one type of microRNA, the cell may bring in another to take its place. This redundancy makes it difficult to study microRNAs one at a time. In addition, even if eliminating a certain type of microRNA does yield interesting results, the effect may be hard to observe. “Many phenotypes are extremely difficult to assay and understand,” Nicoli said. “With miR-107, for example, the fish looked completely normal from the outside.”
For Nicoli, though, the daunting complexity of microRNA only makes it all the more appealing. “I have always liked finding the answers to very complicated biological questions,” she said. In fact, to study microRNA more efficiently, Nicoli has already launched her next project — knocking out different combinations of microRNA using a technology called CRISPR-Cas9. “We’re conducing a genetic screen on noncoding RNA to see which ones are really important,” Nicoli said. “It’s a big project, but it’s the only way to assay what they’re doing.”
By studying miR-107 and other players in the microRNA world, Nicoli and her colleagues have just begun to unravel the mysteries of development. According to Nicoli, we have barely scratched the surface when it comes to understanding microRNA — and that is exactly what makes the field irresistible. “Even with 15 years of study behind us,” she said, “I think we still have many things to do.”
Note: Dr. Nicoli is currently looking for undergraduate research assistants to join her lab. Please contact stefania.nicoli@yale.edu for more information.
About the Author: Rebecca Su is a junior biomedical engineering major in Silliman College. She has previously served as Editor-in-Chief and Features Editor of the Yale Scientific Magazine.
Further Reading: Ristori E, Lopez-Ramirez MA, Narayanan A, Hill-Teran G, Moro A, Calvo CF, Thomas JL and Nicoli S: A Dicer-miR-107 Interaction Regulates Biogenesis of Specific miRNAs Crucial for Neurogenesis. Dev Cell. 2015 Mar 9;32 (5) :546-60. Epub 2015 Feb 5. PMID: 25662174
Cover Image: Art by Hannah Yang.
Acknowledgements: The author would like to thank Dr. Stefania Nicoli and Emma Ristori for their time and dedication to their research.