Anopheles gambiae is professor Richard Baxter’s insect of interest, and it is easy to see why: The mosquito species found in sub-Saharan Africa excels at transmitting malaria, one of the deadliest infectious diseases. “[Malaria] is a scourge of the developing world,” said Baxter, a professor in Yale’s chemistry department. Discovering a cure for malaria starts with understanding its most potent carrier.
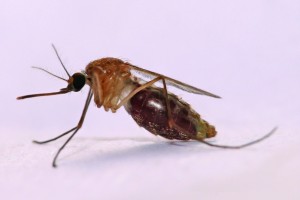
This is one research focus of the Baxter Lab, where scientists are probing the immune system of A. gambiae mosquitoes for answers. Despite being ferocious in their transmission of malaria to human populations, these insects show a remarkable immunity against the disease themselves. With ongoing research and inquiry, scientists could one day harness the immune power of these mosquitoes to solve a global health crisis — rampant malaria in developing countries.
The story of Baxter’s work actually starts with a 2006 study, a pioneering collaboration led by professor Kenneth Vernick at the University of Minnesota. Vernick and his team collected and analyzed samples of this killer bug in Mali. The researchers were surprised by what they found. Not only did offspring infected with malaria-positive blood carry varying numbers of Plasmodium, the parasite responsible for transmitting malaria, but a shocking 22 percent of the mosquitos sampled carried no parasite at all.
Since then, scientists have turned their attention to the complex interplay between malaria parasites and A. gambiae’s immune system. Vernick’s group correlated the mosquitos’ genomes with their degree of parasite infection, and identified the gene cluster APL1 as a significant factor in the insect’s ability to muster an immune response.
Now, nearly a decade following Vernick’s research in Mali, A. gambiae’s immune mechanism is better understood. Three proteins are key players in the hypothesized immune chain of response: APL1, TEP1, and LRIM1. TEP1 binds directly to malaria parasites, which are then destroyed by the mosquito’s immune system. Of course the molecule cannot complete the job alone. TEP1 only works in combatting infection when a complex of LRIM1 and APL1 is present in the mosquito’s blood and is available as another line of defense.
To complicate matters, this chain of response is a mere outline for the full complex mechanism. Gene cluster APL1 codes for three homologous proteins: to put it simply, these proteins can be categorized as A, B, and C. According to Baxter, this family of proteins may serve as “a molecular scaffold” in the immune response. Though they all belong to the APL1 family, each individual protein may serve a distinct purpose within A. gambiae’s immune system. Herein lies one of Baxter’s goals — uncover the functions and mechanisms of the individual APL1 proteins.
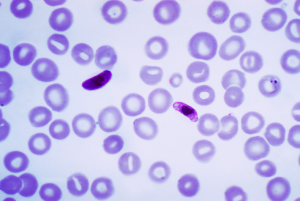
Prior research has elucidated the role of protein C in this family. Scientists have observed that LRIM1 forms a complex with APL1C, and this complex then factors in to the immune response for the mosquito. How proteins A and B contribute to the immune response was poorly understood.
In Baxter’s lab, confirming whether LRIM1 forms similar complexes with A and B posed a challenge, namely both proteins show instability. After experimenting with different approaches, Baxter’s team found that LRIM1 does indeed form complexes with APL1A and APL1B. The scientists also observed some binding with TEP1, the protein that attaches itself directly to the malaria parasite, which could further explain how this mosquito’s immune system puts up such a strong shield against malaria.
Protein B in the APL1B family still presents the most unanswered questions. Previous studies have shown that APL1A expression leads to phenotypes against human malaria, and APL1C to phenotypes against rodent malaria. The role of APL1B remains cloudy. “Being contrarian people, we decided to look at the structure of APL1B because it’s the odd one out,” Baxter said.
His lab discovered that purified proteins A and C remain monomers in solution, while APL1B becomes a homodimer, or two identical molecules linked together. Brady Summers, a Yale graduate student, went on to determine the crystal structure of APL1B. This focus on tiny molecules is all motivated by the overarching, large-scale issue of malaria around the globe. The more information that Baxter and other scientists in this field can learn in the lab, the closer doctors will be to reducing the worldwide burden of malaria.
“Vast amounts of money are spent on malaria control, but our methods and approaches haven’t changed a lot and are susceptible to resistance by both the parasite and the mosquito vector,” Baxter said. It is crucial, then, that research continues to shed light on the structure and function of immune complexes in A. gambiae. A better understanding of A. gambiae in the lab is the first step towards developing innovative, effective measures against malaria in medical practice.
Cover Image: The model shows the LRIM1/APL1C protein complex, found using small angle X-ray scattering (SAXS). Image courtesy of Professor Richard Baxter.