An estimated 0.7 percent of power plants today use nuclear power to sustain a whopping 5.7 percent of the world’s energy and 13 percent of the world’s electricity. Despite the clear importance of nuclear power plants, they do not operate without risk. Indeed, on-site explosions can release radiation equivalent to that of multiple atomic bombs — radiation that persists, seemingly without end, for thousands of years.
Though nuclear plant explosions are uncommon, several have occurred in recent decades. One of the most infamous examples occurred in 1986, when a nuclear reactor exploded in Chernobyl, spewing massive amounts of radioactive material into the atmosphere. Shockingly, scientists estimate that this explosion alone released a hundred times more radiation than the atomic bombs dropped on Hiroshima and Nagasaki.
The explosion at Chernobyl is well known, but what is less clear is exactly what makes radioactive matter so deadly. Radioactive materials often come in the form of high-energy photons, or tiny packets of energy that can permeate through matter impermeable to ordinary, less-energetic photons. Human health is at stake when radioactive elements work their way into cells. This dangerous material can cause deaths, deformities, and cancers when it encounters bodily tissue, depending on the type and amount of radiation released. The infamous Chernobyl disaster, the deadliest unintentional release of radioactive matter in history, accounts for just shy of one million deaths to date.
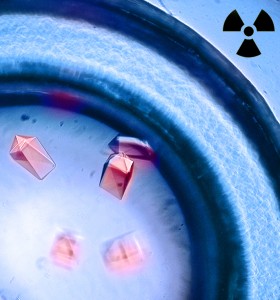
Until recently, scientists have known little to none about how cells take in high-energy radioactive materials. This past July, a team led by Rebecca Abergel of the Lawrence Berkeley National Laboratory in collaboration with Roland Strong of the Fred Hutchinson Cancer Research Center discerned a pathway for the cellular uptake of radioactive matter. With this new insight, the researchers hope to bring a drug counteracting radioactive health effects to the clinic. A solution that assuages the bodily damage caused by high-energy photons would aid those suffering from the aftermath of disasters like Chernobyl. While it may be impossible to eliminate all radioactive catastrophes, the goal for Abergel, Strong, and their colleagues is to find better ways to respond to future disasters.
During nuclear reactions, many heavy elements autonomously emit radiation. The fact that these heavy metals spontaneously spew out photons of energy makes them highly dangerous and intractable. The pathway identified by Abergel’s team concerns heavy metals such as americium and plutonium, classified in a group called actinides. The researchers made a cluster of new discoveries, but most importantly, they determined that a known antibacterial protein called siderocalin is capable of carrying actinides into the cell. They published their findings in a recent issue of The Proceedings of the National Academy of Sciences.
Abergel and her group have a history of achievement in this area. Before their discoveries about siderocalin, they had already developed a molecule to isolate and subsequently remove actinides from the body, which currently awaits approval from the FDA. In a pill, the molecule may help to remove some actinides from the body. However, its efficacy is limited because it works mostly for metals that are still circulating, not for metals that have already been imported into the intracellular space. There was a gap in scientific knowledge of how radioactive elements enter the inside of a cell, and Abergel was determined to fill it. The limitation they noticed in their drug helped motivate the researchers’ efforts to decipher a more mechanistic understanding of how cells are contaminated with radioactivity.
Determining the precise role that siderocalin plays in the cascade of events leading to actinide absorption was a challenging task. The researchers combined experimental techniques spanning different disciplines, from heavy-metal inorganic chemistry to structural biology. They hypothesized that siderocalin might be a good protein to investigate because of its known role in the sequestration of iron, a lighter metal, in the cell. However, they were uncertain whether siderocalin could carry heavier metals such as actinides — no structures of protein-heavy metal ion complexes have ever been cited in scientific literature.
But the team hypothesized correctly, and found that siderocalin can indeed transport metals heavier than iron. First, Abergel’s group created crystals that each contained many identical snapshots of siderocalin in the action of carrying an actinide ion. Next, the team took its crystals to the Advanced Light Source, a synchrotron X-ray source owned by the Department of Energy and located at Berkeley Lab. There, the researchers fired X-rays — high-energy photons — at their crystals. Because the wavelength of an X-ray is approximately the distance between the atoms in these crystals, X-rays were unable to pass through untouched. Instead, they were bent, or diffracted, by the varying electron densities at different points in the crystal. The extent to which these rays were bent created what is known as a diffraction pattern that contained an abundance of exploitable information about the crystal’s structure.
With further mathematical analysis of their diffraction patterns, Abergel and her team inferred the original regions of high and low electron density in their crystals. From this data, the group constructed atomic models that specify the original structures of siderocalin attached to different heavy metal ion complexes. These atomic models somewhat explain the mechanism for cellular uptake of actinides. In general, the structures suggest that first, smaller molecules recognize actinides in the cell and form complexes around the heavy metal ions. Then, siderocalin recognizes these complexes and shuttles them further into the cell to be absorbed.
The group’s discoveries did not stop there. While searching for a mechanism for the cellular uptake of heavy metals, Abergel and her team also found a way to readily identify the presence of these metals in vitro, or in a test tube rather than a living cell. It was truly a testament to science and serendipity. The researchers discovered that the crystals they originally prepared actually luminesced under exposure to ultraviolet light. Through a series of follow-up tests, the team demonstrated that siderocalin can also act as a synergistic antenna that causes heavy metals to glow much more brightly than they would if exposed to ultraviolet light in their bare form. This discovery highlights potential applications for siderocalin in the field of bioimaging, which relies on luminescent signals in a variety of scenarios.
With new knowledge about siderocalin and actinides, Abergel’s team hopes to improve the lives of many who have been exposed to radioactive materials.
Cover Image: Art by Hannah Kazis-Taylor.