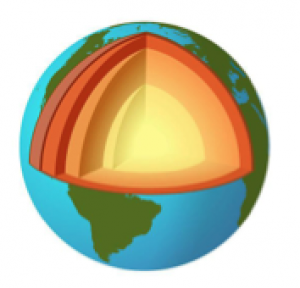
“Bridgmanite” is hardly a household name. And yet, bridgmanite is likely the most abundant mineral on Earth, composing much of the mantle, the thickest layer of our planet. This mineral may provide clues into how the solid Earth—and its atmosphere—has evolved over its long history.
New research from Yale Associate Professor of Geology & Geophysics Kanani Lee and former Yale postdoctoral researcher Tingting Gu suggests that slight differences in the oxidation state of bridgmanite affect its density, and this may have allowed oxidized, less dense bridgmanite to collect near the Earth’s early surface. This phenomenon may have influenced the oxygenation of Earth’s early atmosphere, profoundly changing the history of early life.
The discovery stemmed from an effort to create bridgmanite with differing oxidation states in the laboratory—the first such attempt ever made. Lee’s team synthesized rock comprised of elements common in the mantle, such as magnesium, iron, silicon, aluminum and oxygen. The team combined these elements in the proper proportions, but with one slight adjustment: in order to reflect the heterogeneous degree to which these elements may be oxidized, they varied the ratio of ferric to ferrous iron, a measure of oxidation. The team then transformed these starting materials into the lower mantle phases, bridgmanite being the most abundant, by exhibiting them to the high pressures and temperatures present in the mantle. To the team’s surprise, the two batches of minerals—which were initially quite similar—had significantly different densities under mantle pressures. “When I saw the first set of results, I thought ‘this can’t be right,’” Lee said.
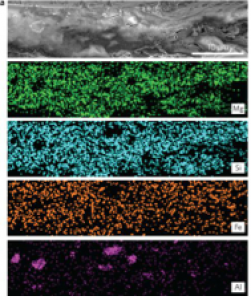
Once the team was confident that the results were robust, they joined efforts with Mingming Li, previously of Arizona State University, to develop a computer simulation to determine the impact of these density differences on the evolution of Earth’s mantle through convection. Stemming from the team’s experimental finding that more oxidized forms of bridgmanite are less dense, the model predicted that an oxidized layer of mantle quickly developed near the surface of the Earth and persisted throughout geological time. With time this oxidized layer was diffused by convection and mixing in the mantle, though heterogeneity in oxidation persists in the model to present day.
The oxidized nature of the upper portion of the mantle may have had profound impacts on the oxygenation of Earth’s atmosphere billions of years ago. “Our model shows all of this oxidized material preferentially towards the surface of the planet, which could affect the composition of volcanic gases that promote more oxidizing conditions at the surface,” Lee said.
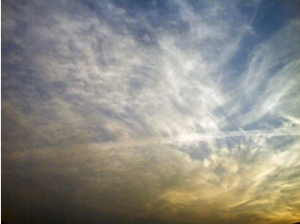
As oxygen was vital to the development of complex life, Lee’s findings may have significant implications on scientists’ understanding of the feedback between early deep-Earth conditions, atmospheric conditions and life itself. Yale Assistant Professor of Geology and Geophysics Noah Planavsky, who studies the oxygenation of Earth’s early atmosphere, said he was enthusiastic about the ability of Lee’s study to illuminate the obscure relationship between the unreachable mantle and events on Earth’s surface. “This study provides the first empirical evidence that if you have a large scale atmospheric oxygen swing, it could potentially be derived from the mantle, as opposed to just Earth-surface processes such as developments in photosynthesis,” Planavsky said. “It makes the question of how the mantle interacts with surface conditions more tractable—it’s no longer entirely speculative,” he added.
While Planavsky’s interest is primarily in the atmospheric implications of this study, Lee plans to focus on the deep end. Lee and her team would like to use these findings to help interpret features observed at the lower portion of the mantle. In addition to looking deep within, she plans to cast her sights afar. These mantle dynamics may be at play on planets other than our own, and could influence the likelihood of life developing on these distant worlds.