Imagine trying to find a needle in a haystack, or a dime in a sea of quarters — the sheer magnitude of the search renders it nearly impossible. That’s what researchers at Yale University and the Salk Institute sought to do when they started their search for extra tiny cellular proteins against the background of the whole proteome, which consists of all of the proteins in the cell.
Researchers at Yale University and the Salk Institute recently developed new techniques for detecting previously invisible microproteins. Their research allowed them to identify 400 new microproteins, amongst which a novel microprotein they called NoBody — short for non-annotated P-body dissociating peptide — proved remarkable due to the role it plays in an important intracellular mechanism. More specifically, NoBody interacts with other proteins in the cell to help clean out excess genetic material in cells.
How a Gene Turns into a Protein
Proteins such as NoBody do not randomly appear in individual cells; rather, these proteins are coded for in each cell’s DNA. In DNA-based organisms, DNA can be thought of as the master computer program that encodes all the different genes, from genes determining eye color to genes determining kidney function. DNA, or deoxyribonucleic acid, is a double stranded helical molecule composed of various nucleic acids bound to a ribose sugar backbone. There are four possible nucleic acids that make up the different bases of DNA: cytosine, guanine, adenine, and thiamine. The four bases can bind to one another to form base pairs, but they always bond in the same pairs, with cytosine binding to guanine and adenine bonding to thiamine. In any given DNA molecule in a cell, the bases on one strand of the molecule are complementary to the bases on the other strand, meaning that they follow the described binding pattern. These chemical interactions allow the DNA to take on the aforementioned double stranded helical shape. These bases are also responsible for encoding all of a cell’s genetic material.
Even though it may seem limiting that cytosine always binds to guanine and adenine to thiamine, it’s really the order in which these bases appear in the genome that matter rather than the variety of bonds they can make. The order of the bases on each strand of DNA code for different genes. Once read and processed, each of these genes codes for a functional protein. The first step in converting the genetic code into a living protein is transcription, the process of converting the gene of interest into a complementary sequence in DNA’s sister molecule, RNA. This RNA resulting from transcription, called messenger RNA or mRNA, codes directly for a protein. The mRNA molecule then undergoes translation, the process that converts the mRNA into a protein. In translation, a cellular organelle called a ribosome reads the bases on the mRNA molecule in three base reading frames called codons. Each of these codons codes for a specific amino acid, used as a building block for generating proteins. As the ribosome translates the mRNA, more and more amino acids are brought over and added to the amino acid chain until the final product, a protein, is formed.
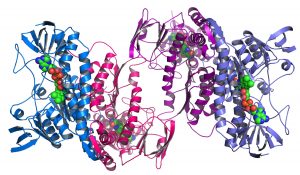
Each protein consists of long chains of amino acids linked together by peptide bonds. The different chemical functional groups on each of these amino acids interact with one another to dictate protein structure and function. The variation in amino acid functional groups allows proteins to take on a wide variety of functions, from catalyzing biological reactions to providing structural support within the cellular cytoskeleton.
NoBody: The Gene Sweeping Protein
NoBody isn’t like most other proteins. For starters, typical proteins can contain hundreds of these amino acids and are incredibly large and bulky. As a result, most protein identifying algorithms are geared towards identifying genes that would code for these long polypeptide sequences. These algorithms are incapable of recognizing shorter amino acid chains and they thus completely overlook the existence of microproteins such as NoBody.
Dr. Sarah Slavoff, an associate professor of chemistry and molecular biophysics and biochemistry at Yale and one of the two senior authors on the paper, first came up with the idea to search for microproteins while still a post-doctoral fellow at Harvard University. “Maybe there are sort of whole classes of human genes that remain invisible to geneticists because they circumvent our expectations of what they look like,” said Slavoff. Current genome sampling algorithms have a strict cut-off as to the length of genes that they can sample, so she had a hunch that most researchers might be under-sampling shorter genes that could yield microproteins such as NoBody. Her collaborator and the other senior author on the paper, Dr. Alan Saghatelian of the Salk Institute, also believed these microproteins might exist, citing previous evidence in flies. As a result, Slavoff and her colleagues set out to develop their own methods to find and catalog these small genes.
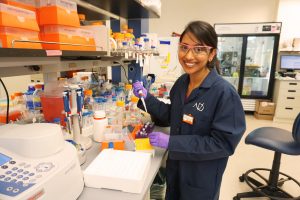
According to Slavoff, the new methods they developed were “conceptually simple,” combining existing methods in large-scale protein analysis and mass spectrometry, an analytical technique that sorts molecules based on their masses. They started their research by breaking apart human myeloid leukemia cells, from which they separated all of the small proteins and peptides. Next, they performed a proteomics work-up of the proteins by enzymatically digesting the proteins and then subjecting them to mass spectrometry to determine the amino acid sequences of the small proteins and peptides. They then compared the amino acid sequences to RNA sequence databases. Any known peptide sequences that matched up with the RNA database were scrapped, leaving the research team with a new database of about 400 novel microprotein sequences.
Out of the 400 newly discovered sequences, researchers decided to focus on NoBody because the protein seems to be highly conserved amongst mammals, despite the fact that it is probably a relatively recent evolutionary development. This high degree of evolutionary conservation suggested that it might play an important role in cellular mechanisms. NoBody, it turned out, was an essential component of the mechanism responsible for mRNA degradation, as it interacted with special clusters of proteins called P-body granules. These P-body granules interact with mRNA to remove the RNA’s protective 5’ cap. Removing the cap initiates the degradation of the mRNA from the 5’ end to the 3’ end. When large amounts of NoBody are present in cells, NoBody interacts with the P-body granules to actually inhibit this, slowing down the general rate of mRNA degradation. In the absence of NoBody, mRNA degrades much more quickly. The degradation of mRNA is important in controlling protein levels in the cell and in making sure the right proteins are created at the right time. Without such a mechanism, each mRNA would constantly be being translated even if the function of the resulting protein is no longer necessary, leading to an overflow of proteins that could kill the cell.
Yet it seems dubious that a protein as small as NoBody is powerful enough to cause such a large change in a cellular pathway. After all, fewer amino acid functional groups largely means fewer possible chemical functions. Additionally, NoBody doesn’t even have a three dimensional structure, taking on a denatured form. However, that doesn’t mean that NoBody is absolutely helpless. According to Slavoff, while NoBody might be too small to perform functions such as catalysis, it can bind the various proteins inside the P-body granules and cause allosteric changes that prevent those proteins from executing their function.
The Future of NoBody
The implications of finding a protein such as NoBody expand the horizons of cell biology, biochemistry, and medicine. For starters, it demonstrates that there is a whole class of previously undetected proteins operating in cellular mechanisms that scientists thought that they knew very well. “[Microproteins] represent a new class of molecule that biologists can explore in health and disease,” said Saghatelian Like other microproteins, NoBody itself can also have a tangible role in human disease. “You can think about [mRNA degradation] as the back end of gene expression regulation,” said Slavoff. This implies that mRNA degradation is another way to regulate the creation of proteins. “This is really important in diseases that have a heavy mutation load such as cancer.” That is because proteins such as NoBody affect the rate at which mutated mRNAs coding for defective proteins are degraded. Increasing the speed of degradation for these mRNAs could stop a cancerous cell in its tracks.
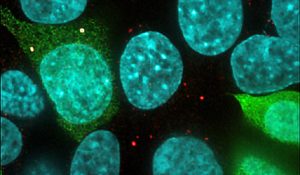
Additionally, NoBody might play a large role in neurological disease. In diseases of aging such as Alzheimer’s disease and Parkinson’s disease, newly translated proteins have a tendency to misfold and create damaging protein aggregates. The proteins in P-bodies can also misfold and create protein conglomerates that may play a role in these diseases. Since NoBody is able to exert a large effect on the structure and function of these P-body proteins, it might also play a role in neurological disease.
There’s still a lot of research to be done on NoBody and microproteins in general. One goal is to gain a better understanding of what are NoBody’s targets in the cell as well as to gain a better understanding of the biochemical interactions that allow NoBody to control the formation of P-bodies. Additionally,Slavoff is working on developing new technologies for identifying microproteins, specifically technology that could potentially combine typical mass spectrometry experiments with experiments determining the function of newly discovered microproteins. Saghatelian’s lab is continuing to characterize the other microproteins, saying, “so far, we have about 400 to study.” Who knew there was so much work to be done on proteins so small in size!
About the Author: Stephanie Smelyansky is a sophomore in Timothy Dwight College studying molecular biophysics and biochemistry. She is the president of Synapse and a member of the Yale Glee Club.
Acknowledgements: The author would like to thank Professor Slavoff and Professor Saghatelian for their time and enthusiasm about their research.
Further Reading: D’Lima, Nadia G., Jiao Ma, Lauren Winkler, Qian Chu, Ken H. Loh, Elizabeth O. Corpuz, Bogdan A. Budnik, Jens Lykke-Andersen, Alan Saghatelian, and Sarah A. Slavoff. “A human microprotein that interacts with the mRNA decapping complex.” Nature Chemical Biology 13 (December 5, 2016): 174-80. December 5, 2016.
Featured Image: A series of labeled test tubes containing samples for various experiments. Image courtesy of the Slavoff Lab.