For many years, gene editing has been hailed as the future of medicine. As the genetic basis of disease becomes clearer, researchers continue to discover more ways to alter the genome and prevent or cure diseases. Recently, a new gene editing tool called CRISPR/Cas9—which involves short, repetitive segments of prokaryotic DNA known as clustered regularly interspaced short palindromic repeats (CRISPR) and Cas9, a nuclease that cuts DNA—has become the primary vehicle for gene manipulation. With any luck, the CRISPR/Cas9 interference may be used in genetic research, a variety of therapies, and even the manipulation of ecosystems.
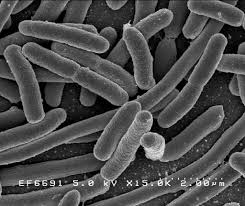
While these clustered repeats were discovered in 1987, their function was not understood until the early 2000s, when researchers discovered that these segments of prokaryotic DNA are a form of immunity for bacteria. They store information about previous viral infections, allowing bacteria to selectively recognize, cut, and degrade foreign DNA. Since its discovery, CRISPR/Cas9 has become a genetic editing tool due to its ability to selectively cut DNA. Researchers have altered the natural CRISPR system to recognize sequences more specifically and in cell cultures and eukaryotic organisms, like yeast.
The CRISPR gene-editing model works by inducing breaks in both strands of DNA. Short pieces of RNA create duplexes, double-stranded RNA molecules that guide Cas9 to very specific locations in the DNA. In nature, these RNA duplexes originate from DNA repeats in the genome, but in the laboratory, these pieces of foreign RNA can be artificially introduced into a cell, allowing the nuclease to find a specific site in the host DNA. These RNA guides can target a DNA sequence up to 20 nucleotides long, providing great specificity since the mathematical likelihood of that 20-nucleotide sequence appearing elsewhere in the genome is very small. After Cas9 cuts the DNA, the double-strand breaks can induce repair pathways that give gene mutations, insertions, or knockouts. By using CRISPR to selectively target sequences, these repair pathways are manipulated to specifically alter the genome. Today, sequence limitations and issues with off-target effects—editing at untargeted sequences due to imperfect specificity—keep CRISPR from widespread use, but many researchers are focused on improving the system. Hopefully, CRISPR/Cas9 will soon be a viable treatment option for a range of genetic diseases.
One recently proposed and unique application of CRISPR/Cas9 is to alter the sex of organisms, specifically mosquitoes. In 2015, a team led by Zhijian Tu and Zach Adelman studied the mosquito species Aedes aegypti and discovered the genetic basis of sex: a male-determining factor transcribed from the gene Nix, which they called the M factor, located on the mosquito’s Y chromosome. “From the perspective of basic research, we are now in a better position to decipher the molecular pathway controlling sex determination in mosquitoes,” said Tu.
The researchers hope to use their newfound understanding of mosquito sex-determination for genetic manipulation. Altering gender ratios within mosquito populations may largely benefit global public health, since , only female mosquitos are responsible for the transmission of blood-borne pathogens. Female mosquitos must feed on blood to acquire protein and produce eggs, but males can survive on nectar and fruit juices. This is true for all mosquitoes, but the Aedes aegypti species was chosen for research because its genome can be manipulated and it is a common vector for dengue, chikungunya and Zika viruses. Together these diseases account for hundreds of deaths per year in Africa, Asia, and the Indian subcontinent. The dengue virus alone affects 2.4 million people each year, causing rashes, high fever, and muscle pain.
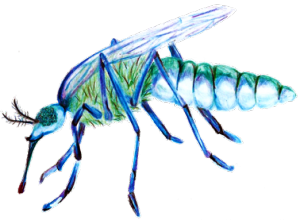
Current research is limited to the Aedes aegypti species, yet other mosquitoes also transmit dangerous, vector-borne diseases. For example, only mosquitoes of the Anopheles genus carry malaria. However, the researchers stress the versatility of their discovery: “The approach that successfully identified Nix may also be used to uncover the M factors in other mosquitoes or insects,” said Tu.
Genetic manipulations that achieve female-to-male conversions or female-lethality might grant researchers partial control of the mosquito population. Methods already exist to reduce mosquito populations, mostly involving insect sterilization, but they are costly and inefficient. The mass production and release of male mosquitoes is likely more efficient and cost-effective than sterilization. According to Tu, it will achieve population reduction and disease control, but it will also have the benefit of male-bias in subsequent generations. This male-bias may prevent the spread of deadly diseases like dengue fever, yellow fever, malaria, and the Zika virus for generations.
These researchers were not the first to consider a male-determining factor in mosquitoes, but they were the first to prove its existence. “The most important result of this research is the discovery and functional demonstration of a male-determining factor, Nix, in mosquitoes. Nearly 70 years ago, it was known that such a master switch gene existed in mosquitoes, but its identity remained elusive despite strong interest,” said Tu. To discover Nix, the researchers designed and used an algorithm, a procedure for solving a problem, that could effectively identify the male-specific DNA sequences.” After it was identified, they demonstrated that Nix was the male-determining factor by showing that it was necessary and sufficient to initiate male mosquito development. By manipulating the factor, they could change a mosquito’s observable sex: overexpression of the M factor masculinized females, and deletions—obtained using CRISPR/Cas9—feminized males.
Later, in a 2016 issue of Trends in Parasitology, the same authors published a paper demonstrating their ability to manipulate the M factor and other intermediary factors, using CRISPR/Cas9. Two targeted transcription factors were Dsx and fru, since they undergo sex-specific splicing events and help determine sex differentiation. By targeting these factors with the CRISPR/Cas 9 system, the researchers could convert females to males, allowing for sex separation and a reduction of the female population. This result builds on the earlier discovery and manipulation of the M factor to drive sex change.
While the benefits of gender targeting in mosquitoes appear obvious, the future of CRISPR/Cas9 remains unclear. Researchers are unsure if these alterations will effect Aedes aegypti genome stability in the long term or if gender manipulations will have unforeseen effects on the ecosystem. CRISPR/Cas9 has already been fraught with controversy over its ethicality. Certain uses of CRISPR/Cas9 are highly contested, for example, should CRISPR/Cas9 be used to create “designer babies,” or is that pushing the boundries of what our society allows? Someday soon, deciding whether to genetically manipulate our ecosystems may be an equally controversial conversation. “CRISPR technology has the potential to significantly improve our health and environment. But we should also be mindful of the potential for misuse and proceed with appropriate risk assessment and regulation,” explains Tu. As science advances, we must consider what applications of CRISPR/Cas9 are prudent and what pushes the boundries too far.