Imagine all of the mass of the earth, the Sun, and the planets, condensed into a single, dimensionless point, a singularity. The object is so unimaginably dense that space-time curves upon itself. Even light, a massless particle-wave, is unable to escape the incredible gravitational force.
Black holes have inspired the imaginations of countless scientists, students, and writers ever since they were theorized in the nineteenth century. Dr. Jeffrey McClintock, a Senior Astrophysicist at the Harvard-Smithsonian Center for Astrophysics, confesses, “to me, [black holes] are the most profound question in the universe apart from human consciousness.”
Distant and foreign objects such as the sun and neutron stars display certain characteristics that indicate some truth about the object. Black holes, however, are completely different. A black hole is “the complete gravitational collapse of matter to a point. That’s profound, in the sense that it’s beyond [any physical] knowledge or comprehension.”
Black holes represent an extreme and completely alien situation in the universe. Information cannot be transmitted from within a defined distance of a black hole (the event horizon) to the outside world. Thus, to study these objects, astronomers must first find, then observe their interactions with normal matter at radii approaching the event horizon.
Jerome Orosz (Ph.D. ’96), who is now an assistant professor of astronomy at San Diego State University, led a group of scientists that studied light from certain compact x-ray sources to search for black holes. They stumbled across M33 X-7, a black hole in a binary system with unique and perplexing characteristics that challenge even our rudimentary theories about black holes.

But what is a black hole?
Black holes were first predicted in 1939 as a consequence of Einstein’s theory of general relativity. General relativity proposed that gravity was the consequence of the curvature of space-time by an object’s mass.
Imagine a bowling ball resting on a taught rubber sheet. The rubber is warped in the vicinity of the bowling ball, causing moving objects that would otherwise follow a straight path to actually follow a curved path without even interacting with the object creating that disturbance. The equations associated with this theory can predict the existence of an object so massive that it would create a gravitational field so strong that not even light could escape.
There are three main types of black holes: micro, stellar-mass, and supermassive black holes. Micro black holes are predicted by quantum mechanics, and supermassive black holes can be found in the center of galaxies, spanning many light-years across.
Smaller, stellar-mass black holes can be found in our own galaxy as well as neighboring galaxies. These are seven to ten times as massive as our own Sun, and are formed from the core collapse of a dying star.
Once all the fuel in a star is exhausted, the core contracts to maintain a balance between the gravitational pressure of the outer layers pressing inwards and that of thermonuclear reactions pushing outwards. As no fuel is present, the inward gravitational force prevails, causing the core to collapse until all has been compacted into a single point of infinite density.
Due to the extreme conditions during the formation of black holes, collapsing stars are stripped of almost all their previous characteristics. As McClintock describes, “all of the ‘hair,’ the magnetic fields, the strange properties of the falling material, all the shockwaves, all gets radiated away in gravitational waves. All you end up with is this pit in space-time and all you need to describe it is [essentially] two numbers.”
Mass and rotational velocity are all that is needed to completely define a black hole. The mass of the compact object being orbited can be calculated using the red- and blue-shifts, measurements of the distortion of light taken as the star moves away and towards us in orbit. The mass obtained through these measurements determines whether the compact object is a black hole.
The rotation of a black hole can be calculated through precise measurements near the black hole’s surface, the event horizon. For a specific rotational velocity, there is a defined radius outside of the Schwarzschild radius (the distance at which even light is pulled into the black hole) at which any material will also fall into the black hole. As the rotational velocity increases, this radius decreases until it reaches a maximum velocity and the inner region of the accretion disc meets the event horizon.
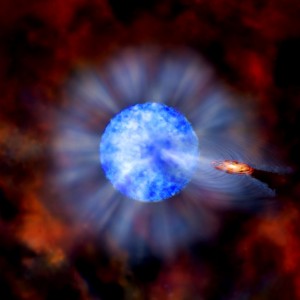
How do you find a hole?
Since information cannot be retrieved from within a black hole, they are usually discovered through their interactions with nearby visible matter. In the case of stellar-mass black holes, they are usually found in X-ray binary systems.
X-ray binaries are systems of two objects: an optical star and a compact X-ray source. A compact X-ray source could be a neutron star or a small black hole. Long after a star in a binary system collapses and becomes a black hole, its strong gravitational field strips and captures gas from the outer layer of the companion star, creating an accretion disk. As the material orbits the black hole at ever increasing velocities, the material releases radiation detectable in the X-ray spectrum.

Two problems must be considered to obtain the mass and rotational velocity of a given black hole in an X-ray binary system: the orbital inclination of the binary and the differentiation of varying sources of emission.
Sources within an X-ray binary emit light that has been distorted in different ways. Accretion is variable, and even when the accretion flow seems to stop and the X-rays fade, some material is still drawn from the companion star into the outer layers of the accretion disk.
At these lower velocities, the material emits radiation in the visible spectrum that could interfere and taint the optical light from the companion star. Professor Charles Bailyn, DUS of the Yale Astronomy Department, explains, “It’s not just separating emission from the accretion flow, but you need to figure out where in the accretion flow you’re looking when you see any given source of emission.”
This issue is important in determining the system’s orbital inclination, the angle at which the orbit is observed. The companion star is distended by the black hole’s gravitational field. If the system is viewed face-on, the visible surface of the star will remain constant, and the brightness will not vary. If the system is viewed edge-on, the star will display both maximum and minimum surface area twice. The lightcurve, or the star’s apparent brightness over time, is used to determine the orbital inclination.
But why is the inclination important? The orientation of the orbit determines the observed red- and blueshift in the light from the optical star. If exactly edge-on, these values will correspond to the actual radial velocity of the star. At any other inclination, however, the derived radial velocity will be lower than the actual value. Through Kepler’s laws and Newtonian mechanics, the velocity found with this angle determines the mass of the black hole.

What is unique about M33 X-7?
A few characteristics distinguish M33 X-7 from the twenty other black holes found in X-ray binary systems. First, this is the first X-ray binary found outside the Milky Way. Its location makes the measurement of the distance to this system far more precise than the distances to previously discovered black holes.
In addition, M33 X-7 is the only system with an orbital eclipse: at certain points, the compact X-ray source is obscured by the companion star. The X-ray output can be sampled to produce a graph that clearly indicates the duration of the eclipse. Because of the precise data from this orbit, the orbital inclination and radial velocities can be accurately calculated.

The third and possibly most intriguing feature of this black hole was its mass. Whereas 19 of the 20 black holes so far discovered have masses between seven and ten solar masses, this system weighs in at more than 15 solar masses. Such a large specimen calls for the reevaluation of standing theory associated with the formation of black holes.
Bailyn explains, “The idea is that those core regions were the ones that collapsed and the hydrogen-rich envelopes were spewed into space. Now it appears that something must happen whereby some of the hydrogen-rich original material actually falls back down into the hole.”
The combination of these unusual characteristics is more important than the mass of the black hole. The black hole is larger than all others found before, the companion star is about 70 solar-masses, and the orbital period is about 3.45 days. According to the study, these unusual properties require a “common-envelope” phase, different from those in standard theory, during which the outer layers of the companion and the collapsing star shared a single surface.
Given the required model for the “common-envelope” for M33 X-7, the two stars should have actually merged. This system has challenged what had previously been standard models for stellar evolution and the creation of X-ray binaries.

What’s next?
Studies that accurately determine the mass of small black holes combined with those from McClintock’s research group that determine the rotational velocity help create a catalog of defined black holes. Bailyn says that the a long-term goal for this research is to understand the behavior and dynamics of the black hole, as “right down there near the black hole, you have very strong relativistic effects that you can’t set up in any lab, so you can test strong-field relativistic effects, but only if you understand exactly what’s going on.”
The discovery of M33 X-7 marks a great development in the observational capabilities of modern astronomy in observing distant small black holes. The search will continue for more of these X-ray binaries, and with this leap in ability, we will be able to find a greater number of these systems.
The more we know about these systems, the more we will be able to probe some of the most mysterious and exotic phenomena in astrophysics. Though we may never solve the mysteries of the human consciousness, this research is drawing us closer to an understanding of intrinsically unimaginable objects.
About the Author:
Michele Dufault is a freshman in Saybrook College. She is considering a major in Astrophysics or Biochemistry.
Acknowledgements:
The author would like to thank both Professor Charles Bailyn and Dr. Jeffrey McClintock for their time and patience in answering all of her questions. She wishes them luck in their continued research in this field.
Bibliography
Bailyn, Charles. “Black Holes Toolbox.” Yale University, Department of Astronomy. https://cmi2.yale.edu/bh/index.html (accessed 14 November 2007).
Freedman, Roger and William J. Kaufmann. Universe, 8th ed. New York: W. H. Freeman, 2007.
Hynes, Rob. “Homepage for Rob Hynes: Assistant Professor in Multiwavelength Observational Astronomy.” Louisiana State University. https://mintaka.sdsu.edu/faculty/orosz/web/ (accessed on 21 November 2007).
Malzac, Julien. “Jet disc coupling in black hole binaries.” Astrophys Space Sci 311, 149-159 (9 August 2007) https://www.springerlink.com/content/e5345x1l34r10038/(accessed 21 November 2007).
Orosz, Jerome A. “Homepage for Jerome A. Orosz.” San Diego State University. https://mintaka.sdsu.edu/faculty/orosz/web/(accessed on 21 November 2007).
Orosz, Jerome A., Jeffrey E. McClintock, Ramesh Narayan, Charles D. Bailyn, Joel D. Hartman, Lucas Macri, Jiefeng Liu, Wolfgang Pietsch, Ronald A Remillard, Avi Shporer, and Tsevi Mazeh. “A 15.65-solar-mass black hole in an eclipsing binary in the nearby spiral galaxy M 33.” Nature 449, 872-875 (18 October 2007), . https://www.nature.com/nature/journal/v449/n7164/full/nature06218.html (accessed 20 November 2007).
“Photo Album: More Images of M33 X-7.” Chandra X-Ray Observatory. https://www.chandra.harvard.edu/photo/2007/m33x7/more.html (accessed on 20 November 2007).