Dark matter? Particle accelerators? Higgs boson? Particle physics has left the public fascinated, and perhaps puzzled, by its potential implications. Current work on an obscure particle called the neutrino may leave even physicists grasping for answers. Researchers at the Yale Wright Laboratory led by professor Karsten Heeger currently design and implement experiments to investigate if neutrinos are a new form of matter. Such a discovery would require a major revision of the Standard Model of Particle Physics.
According to the Standard Model of Particle Physics, neutrinos are neutral, massless elementary particles—matter that cannot be further subdivided. The neutrino can take three forms, the electron, tau, or muon neutrinos, and can only be acted upon by the universal weak force. The Standard Model attempts to explain the interactions in the subatomic world but cannot account for phenomena such as dark matter and dark energy.
The Wright Lab’s research on neutrinos is groundbreaking because it shows that the assumptions of the Standard Model are even more flawed than previously thought. In 2016, Heeger shared the Breakthrough Prize in Fundamental Physics for three experiments showing that neutrinos can change their “flavor” as they travel through space. These changes in flavor—from electron to muon neutrinos, for example—are called neutrino oscillations and show that neutrinos have mass.
“If you weighed all neutrinos in the universe, their combined mass would equal that of the mass of all the visible stars in the sky,” Heeger explained.
Heeger’s group is involved in several neutrino experiments to determine the nature and mass of the neutrino and to search for the existence of a possible fourth form of neutrino—the sterile neutrino. One of these, Project 8, makes inferences about neutrinos based on electrons emitted from radioactive beta decay.
Another project called the Cryogenic Underground Observatory for Rare Events (CUORE) studies a special form of nuclear decay called neutrino-less double beta decay and tests if neutrinos are their own antiparticles. Every particle has a counterpart antiparticle with the same mass but opposite charge; in the chargeless neutrino’s case, a quantum mechanical property called handedness varies instead. Antineutrinos are directly detected from nuclear reactors and indicate the presence of neutrinos. In ordinary double beta decay, two neutrons within a nucleus change into two protons and emit two electrons and two antineutrinos. However, in neutrino-less double beta decay, two neutrons are converted into two protons and two electrons are emitted—no antineutrinos. This is possible only if neutrinos are their own antiparticle.
The Precision Oscillation and Spectrum Experiment (PROSPECT) investigates neutrinos taken from an active nuclear reactor. What distinguishes this project is its short baseline, or distance between the neutrino source and detector—10 meters rather than the usual hundreds. The experiment measures the variation in neutrino flavor—the neutrino oscillation—over short distances. Results could provide evidence for the sterile neutrino, which is unaffected by the weak force and thus an entirely new form of matter.
The discovery of the sterile neutrino would be no less than a “paradigm-shift for the whole [scientific] community,” said Danielle Norcini, a graduate student working on PROSPECT.
Findings about whether neutrinos are their own antiparticles and whether sterile neutrinos exist could require a revision of the long-standing Standard Model of Particle Physics.
“If neutrinos are their own antiparticles, then there has to be a new term in the [Standard Model] that describes how particles get their mass…there has to be more than just the Higgs boson. If we discover sterile neutrinos, then there would have to be a whole new class of matter [added to the theory],” Heeger said.
Aside from its implications in theoretical physics, neutrino research has tangible applications. Beyond the laboratories of experimental physics, advanced forms of neutrino detection would prove valuable to nuclear reactor monitoring. Neutrinos from a reactor core can describe the contents of the reactor, including the type of radioactive fuel used and the type of radioactive decay occurring. Some of the unique advantages of neutrino detection include its harmlessness, as neutrinos do not affect humans physically, as well as the neutrinos’ ability to pass through any barrier unimpeded—no man-made method can hide their presence.
In addition, neutrinos are integral to the grand scheme of the universe as we know it.
“Without neutrinos, supernovae wouldn’t happen. Supernovae are important for producing the elements that we are made of,” Heeger said.
Future Wright Lab research will further the scientific understanding of neutrinos and particle physics with applications that extend into cosmology and astrophysics.
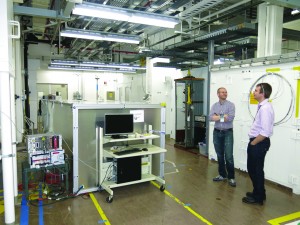