Synthetic biology, an emerging and fascinating field at the crossroads of natural and technical science, once served only as fodder for sci-fi films. And while it’s doubtful that scientists are working on the next Frankenstein, the field has since progressed rapidly: Researchers are now designing and manipulating biological molecules, engineering life forms to do anything from delivering chemicals to producing biofuels.
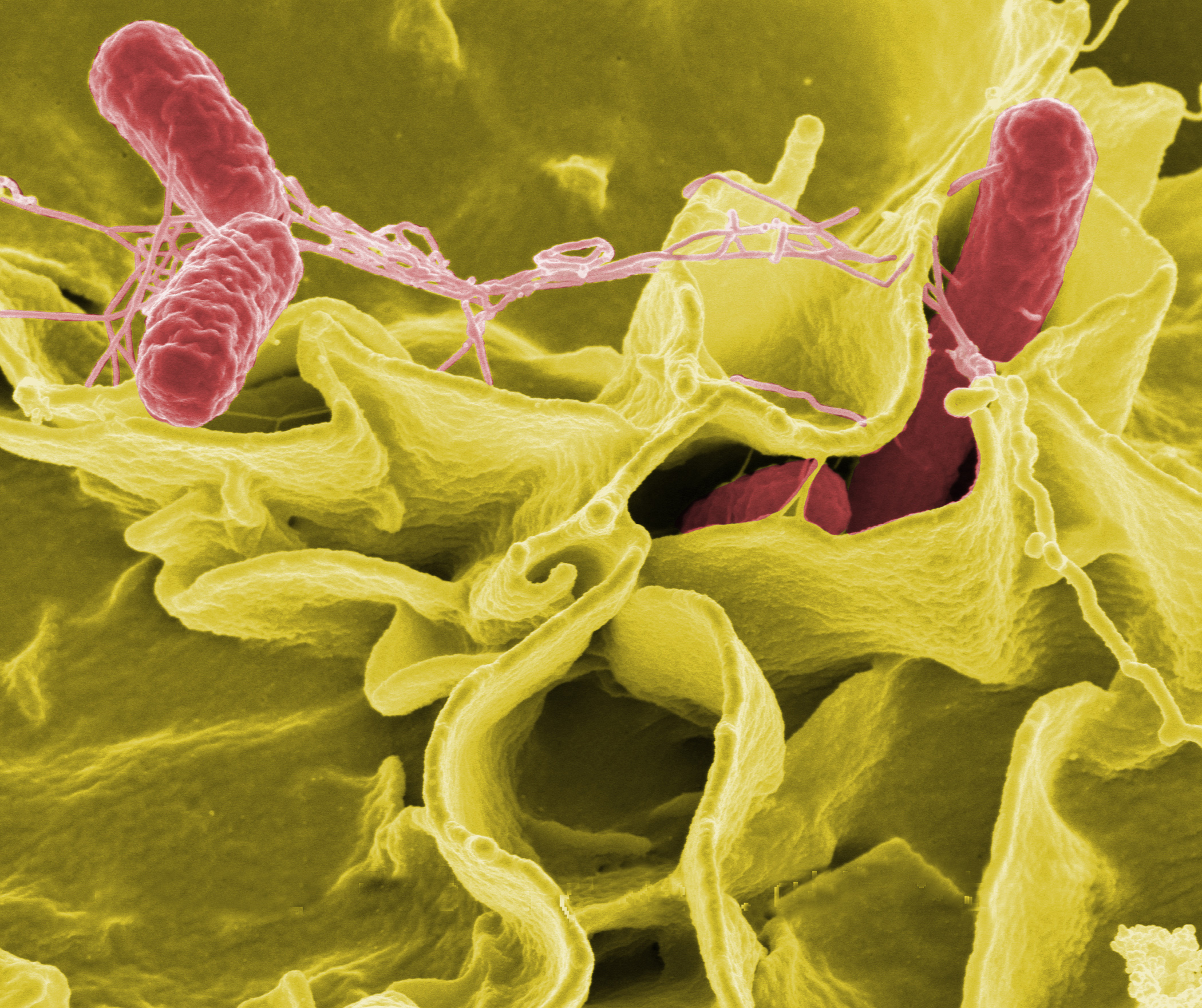
Most recently, a lab at the University of California, San Diego (UCSD) engineered Salmonella, a common pathogenic bacterium often associated with food poisoning, to fight cancer. It sounds counterintuitive, since we usually think of Salmonella as dangerous, but the magic of it lies in the science behind the bacteria’s mode of action.
The researchers used the bacteria to produce and transport a confirmed anti-cancer chemical, Haemolysin E, to cancerous cells. Haemolysin E destroys cancer cells, and mammalian cells in general, by creating pores in the cells to cause them to lyse, or rupture and release their cellular contents. Since the bacteria release the chemical directly to the cancer site, this helps prevent the toxin from spreading to healthy cells. The researchers programmed Salmonella to produce Haemolysin E by inserting a plasmid, or a small piece of circular DNA, with the Haemolysin E gene into the bacterial DNA. The bacteria could then produce the toxin independently and at sufficient quantities.
Haemolysin E is not a newly discovered toxin, but the Salmonella research at the UCSD lab is notable for its use of bacteria as a vector for drug delivery. Jeff Hasty, who heads the lab, and his team chose to work with bacteria because of a fairly recent discovery that bacteria actually live and thrive inside of tumors. Though scientists aren’t entirely certain why this is the case, one theory is that developing tumors allow bacteria to enter through the blood when the tumor is building its system of blood vessels. The researchers concluded that bacteria could effectively transport materials because they naturally localize to tumors, are grown quickly, and can be easily manipulated by genetic engineering. The bacterial strains they used were attenuated to be non-pathogenic and had been tested for safety in human clinical trials.
Once the Salmonella arrive at the treatment site, Haemolysin E must exit the bacteria to make contact with cancer cells. To accomplish this, the researchers programmed the cells to lyse after reaching a minimum population density. They engineered a sensing system within the cells so that when the bacteria reached a certain population density, they released small, diffusible molecules that signaled it was time to lyse. Lysis releases all of the cells’ contents, including the toxin that then acts on the tumor. But the process doesn’t stop there, a small number of unlysed cells remains to repopulate the site and repeat the cycle: they proliferate, reach a high population density, lyse, and recolonize. The bacterial population acts like a clock, cyclically pulsing up to the threshold population and back down again at the appropriate times.
Working out the kinks of this cycle was a major challenge for the researchers, who hope to design a better way to stabilize the circuit. “Ideally, we would want the bacteria to retain the circuit for a long period of time with a reduced risk of mutations,” said Omar Din, a UCSD graduate student who performed the Salmonella research. Bacteria have the potential to mutate quickly, and these mutations can interfere with their function.
To prove their method was actually effective against cancer, the researchers first tested the genetically modified bacteria in vitro, in a culture dish, by growing human cancer cells with the engineered bacteria. The cancer cells died when the bacterial cells were lysed, indicating successful toxin delivery. Next, the researchers tested the agent in mice with liver tumors. Test mice received either an oral dose of bacteria, chemotherapy, or both treatments. Though the bacteria-only treatment wasn’t very effective, mice who received both treatments had less tumor activity and a greater survival rate than those who received either single treatment, suggesting that bacterial treatment is most effective when combined with chemotherapy. Hasty predicts that combined treatment works best because each individual treatment targets a different area. Chemotherapy is most effective in the oxygen-rich environment outside tumors, while the bacteria inhabit the area inside tumors.
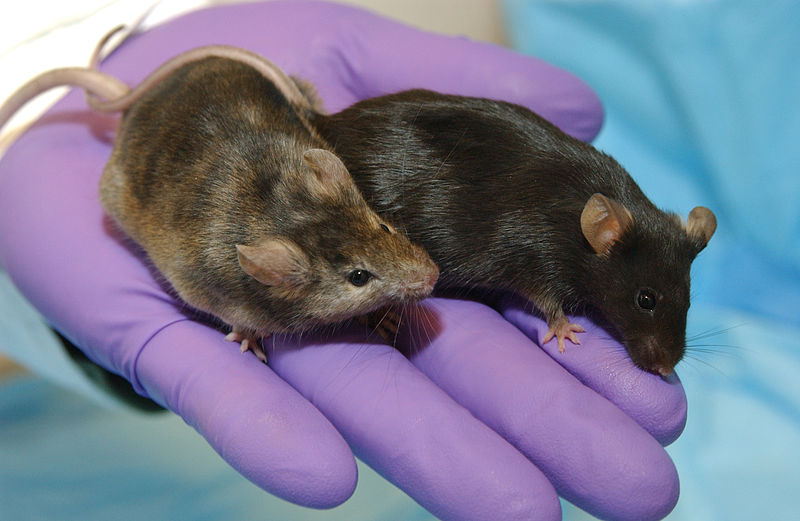
For now, Hasty’s lab found that their engineered Salmonella decreased tumor sizes in mice for approximately twenty days before the tumors began to grow again, significantly increasing the mice’s life expectancies. However, Salmonella research has yet to provide a method for treating cancer in humans, since additional research must first determine the host’s response to the treatment and its long-term effectiveness. The work done in Hasty’s lab is promising because the lysis steps keep the bacterial population low, minimizing potential for a negative host response. Their research sets a precedent for the future of synthetic biology: Hasty’s lab has identified Salmonella as a platform for delivering chemicals to diseased cells, exploiting the bacteria’s tendency to localize and flourish in a cancer-cell environment.
“We hope to highlight the utility of using synthetic biology to engineer bacteria for therapeutic purposes,” said Din. “This will ideally include collaboration between groups working in synthetic biology and cancer research.” Further work with Salmonella may improve the treatment to maximize effectiveness and further prove safety. Once that has been done, scientists can test how best to administer the treatment and at what dosage. If all of those pieces fall into place, bacterial therapy could potentially reach human clinical trials in a few years.
The momentum of bacterial therapy lies in its versatility: it doesn’t stop at cancer. “Bacteria can theoretically deliver any protein or molecule they are capable of producing,” said Din. These molecules could range from toxins like Haemolysin E to the proteins normally produced in mammalian cells. For example, bacteria could be engineered to deliver chemokines, a type of signaling molecule that attracts white blood cells and other immune system cells to an infected site in the body. This case presents an interesting paradox: bacteria can treat an infection instead of causing it.
The Salmonella research at UCSD exemplifies synthetic biology’s ability to engineer living things to synthesize a product or get a job done certainly an improvement from the era of Mary Shelley’s science-fiction masterpiece.